Elevated temperatures, harsh environments, and abrasive materials make corrosion a major cost to the power-generating industry.
Making the right choices in materials and coatings up front and monitoring conditions thereafter could save utilities and their customers millions and prevent downtime.
“Exposure to oxygen-containing environments can drive corrosion of all metals under specific conditions,” says Angelique Lasseigne, Ph.D., CEO of G2MT Labs in Houston, Texas, USA.
She says several types of corrosion are observed in power plants whether the material is steel, stainless steel, or a superalloy:
- High-temperature corrosion mechanisms such as high-temperature sulfidation and oxidation
- Hydrogen cracking and high-temperature hydrogen attack
- Stress corrosion cracking and/or fatigue cracking dependent on the applied load
- Corrosion under insulation and/or crevice corrosion
“These corrosion mechanisms can be controlled and monitored with proper material selection and having an appropriate understanding of the operating conditions, such as temperatures, pressures, and velocities,” Lasseigne explains.
David Simkins, director of engineering and technology for Polygon US, a pioneer and industry leader in the world of dehumidification and engineered temporary climate solutions, says utilities often must select the lowest bidder instead of availing themselves of the best coatings to protect against corrosion.
“Utilities don’t all take advantage of the higher-tech coatings that have a longer service life,” Simkins says. “That would absolutely help them keep these products in service. They're harder to apply, require a little bit more sophistication in terms of application, but the end result is that these great coatings have longer warranties.”
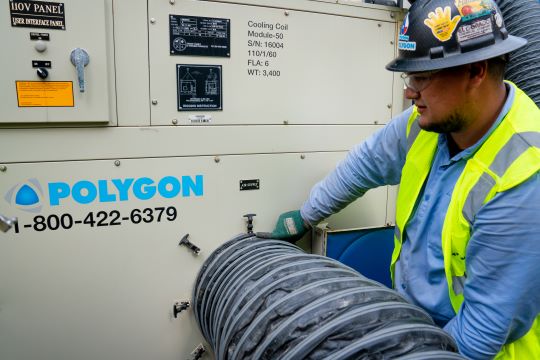
Causes and Costs
In their white paper “Corrosion Reduction in Power Distribution” for the Institute of Electrical and Electronics Engineers (IEEE), researchers Jesse W. Taylor, Ph.D., Kenneth W. McFarland, and Stephanie Ellis note that the highest costs of corrosion are business interruptions that occur when equipment and assets fail to perform as intended.
Design, manufacturing, and construction costs include material selection, protection technologies, and costs related to labor equipment and overhead, they say. Corrosion management-related costs include inspections, maintenance, and repairs due to corrosion damage, as well as material and labor costs related to replacement of corroded components.
Indirect costs include environmental clean-up, litigation costs, and downtime or loss of production.
“For them to take a critical piece of hardware offline has a pretty significant impact on their ability to generate power, and in turn to generate revenue,” says Polygon’s Simkins, who is based in Stuart, Florida, USA and has supported the coatings industry for 32 years. “If that coating fails prematurely and that piece of equipment needs to be taken offline unexpectedly, that's definitely a risk.”
Government Accountability Office researchers reported that by preventing corrosion instead of treating it as it happens, the annual cost of corrosion could be reduced by as much as 40%. Corrosion of transmission towers and poles for electric utilities alone costs the industry $6.9 billion, according to AZZ Inc., an independent provider of hot-dip galvanizing and coil coating solutions.
Most power stations burn fossil fuels such as coal and natural gas to generate electricity, according to the IEEE paper, with nuclear, hydroelectric, solar and wind power gaining in usage. The direct cost of corrosion to the industry, according to the white paper, is estimated to be $27.7 billion.
Lasseigne, whose G2MT Labs provides metallurgical and failure analysis services, says the only way to prevent corrosion of metals is by preventing their exposure of metals to corrosive media, specifically oxygen, through the control of the environment and application of protective coatings.
“Unfortunately, eliminating oxygen and other corrosive media from the environment is often not feasible, therefore advanced coatings provide the barrier between the metal and corrosive fluid eliminating the potential for corrosion damage,” she says.
Coatings have continued to advance over the years, Lasseigne says, with improvements in:
- Bond strength to prevent moisture absorption between the coating and the metal.
- Wear and erosion resistance in systems exposed to turbulent liquids or gases.
- Chemical resistance to even more aggressive environments.
- Operation at higher temperatures. Even the most advanced superalloys are still susceptible to corrosion damage in some aggressive environments and must rely on advanced coatings for safe operation.
The IEEE paper’s researchers report that fossil fuel plants tend to experience corrosion issues when they have buried storage tanks for fuel and underground piping systems, leading to galvanic corrosion because of improper joining of dissimilar components. Electrical equipment buried underground can experience corrosion because of soil content and differences in electrolytes. Coal-burning power plants create ash, which can be corrosive, and systems near the scrubber area can be affected by hydrochloric acid.
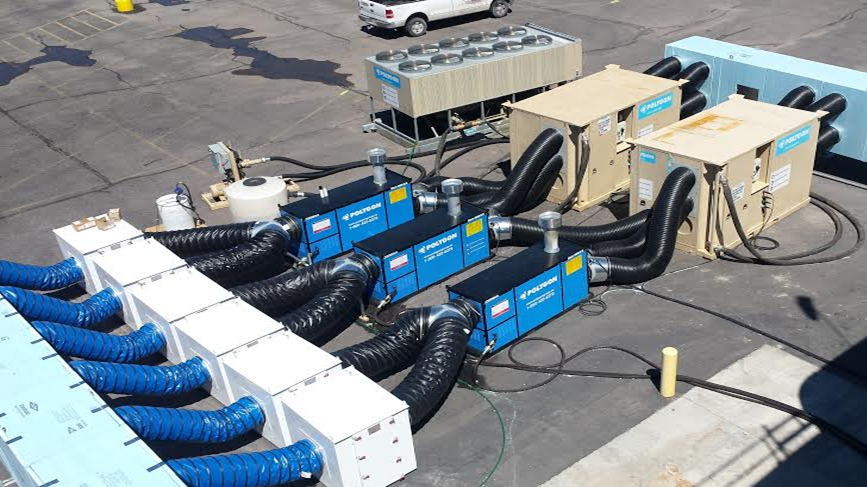
Prevention
“As power plants look to increase efficiencies and lower maintenance costs, corrosion prevention becomes critical,” the IEEE paper’s researchers say.
Polygon’s Simkins and G2MT’s Lasseigne both advocate for the best possible coatings before any power-plant system is put in place.
“Application of a proper coating treatment before a metal component or system is put into service in a corrosive environment will prevent corrosion issues before they start and therefore will provide significant economic benefit over time,” Lasseigne says.
She further explains that if a coating treatment is not applied to an existing system before service and corrosion damage occurs, the existing metal would need to be inspected to ensure lack of corrosion damage in the form of wall loss, pitting corrosion, and/or cracking. Then the metal would have to be repaired/replaced and then appropriately cleaned and coated, which would be much more costly than coating the system beforehand.
“The corrosivity of the fluids/gases in the system should be understood so that appropriate coatings are applied, and corrosion prevented,” she says.
The key, Simkins says, is for utilities to invest in the coat that provides the longest life. He adds that certain coatings have become greener over the years as volatile organic compounds have been reduced, but at a higher cost.
“The return is that it obviously has minimized impact,” he says. “It’s often argued that there’ll be more energy put into the construction of one of these facilities than the energy used in the next 30 years of life. So, in terms of carbon offset, the more that can be done in the construction, in terms of minimizing that environmental impact, has a greater return on the total impact of this structure over time.”
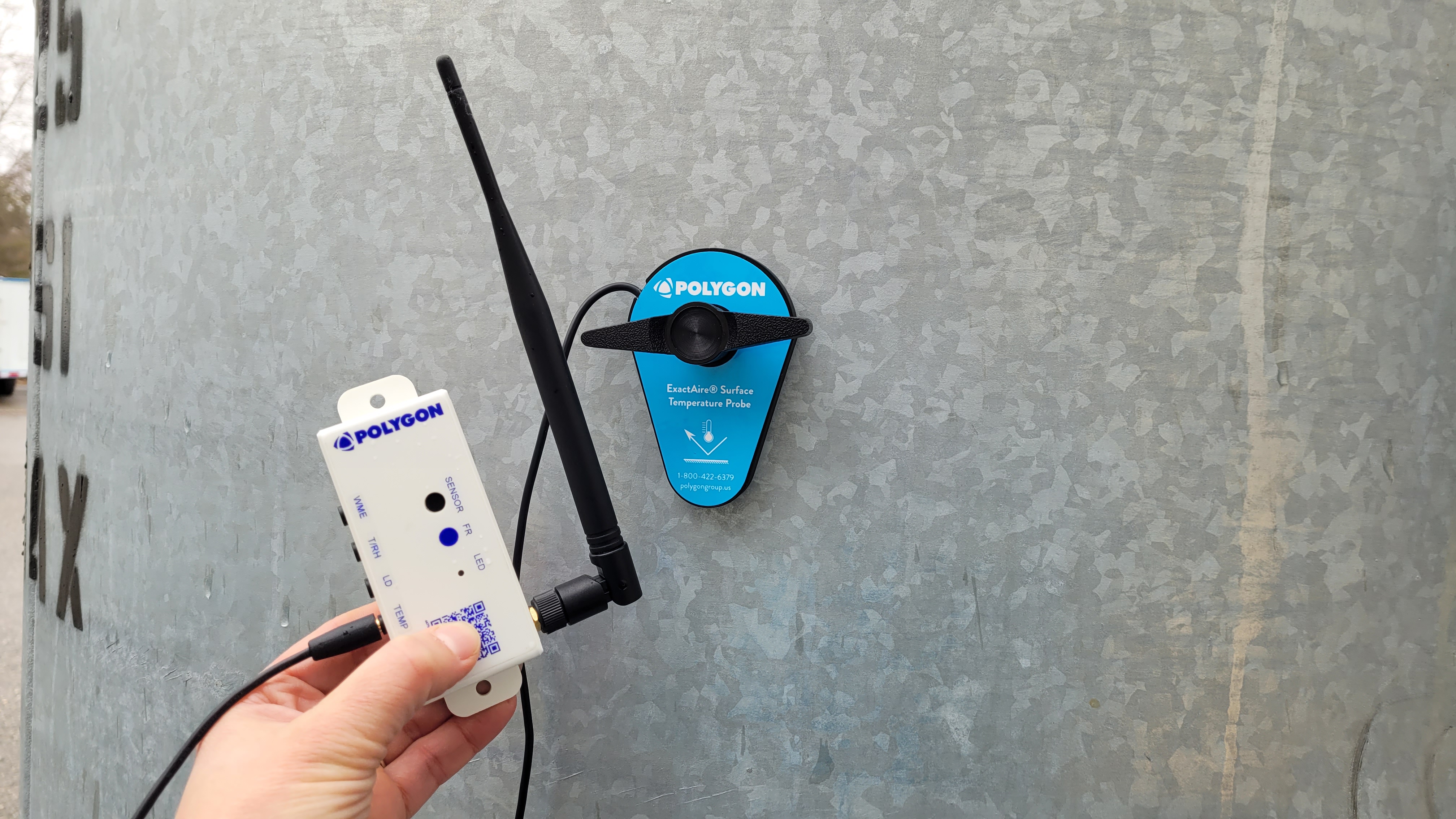
Monitoring
Utility managers looking to prevent downtime and provide the most cost-effective service for their customers should have a corrosion monitoring program that will allow them to understand the failure mechanisms that each component is susceptible to under the service conditions, Lasseigne says.
The monitoring program should be set up to determine:
- The types of inspections that should be performed
- When and how often they should be performed
- Criteria for repair and replacement of parts exhibiting corrosion damage in the form of wall loss, pitting, and other localized corrosion, mechanical damage, or cracking
“Any components that exhibit accelerated corrosion damage should be analyzed to determine the root cause of corrosion to make appropriate actions to prevent the same corrosion damage in the future,” she says.
Whether energy is created by electric, hydro, wind, or nuclear means, a majority of the same materials are used throughout all industries and suffer from similar issues based on the service conditions. These materials include carbon steel, 300 series austenitic stainless steels, duplex stainless steels, high-strength martensitic steels and stainless steels, titanium alloys, and various nickel-based alloys known as superalloys.
“The main variations between industries are the differences in the types of environments of exposure, the corrosivity of the environment, the types of corrosion based on environment, and the types of loads the various components experience,” Lasseigne says.
The nuclear industry, she adds, deals with the most aggressive environments due to radiation exposure, which causes aging and degradation of the exposed metals, including even more advanced alloys such as Zircaloy, made from zirconium. The hydro and wind industries, on the other hand, deal with issues with corrosion fatigue, stress corrosion cracking, and micro-biologically induced corrosion.
“Understanding the potential corrosion risks in each environment is the key to preventing and monitoring corrosion in the future,” she says.
Editor’s note: This article first appeared in the September 2023 print issue of Materials Performance (MP) Magazine. Reprinted with permission.