Rudolf Diesel, the inventor of the compression ignition engine, originally designed the engine to run on vegetable oils. In 1912, he stated, “The use of vegetable oils for engine fuels may seem insignificant today, but such oils may become in the course of time as important as petroleum and the coal tar products of the present time.” However, with the development of low-cost diesel, the utilization of vegetable oils as fuels was relegated. Later, in 1937, the first patent related to the biodiesel production process was granted in Belgium. It was produced by the transesterification of vegetable oils by using ethanol. The main reason to convert the vegetable oil into biodiesel was to reduce its high viscosity and to improve its burning properties.1
Due to the oil crisis in the 1970s, such as energy security and environmental issues, the development of biofuels was encouraged. Hence, once again the use of vegetable oils was brought to the forefront. Currently, the United States and the European Union have the highest shares in global biodiesel production, followed by Argentina, Brazil, Indonesia, Singapore, Thailand, and other countries.2
In Mexico, there is still not a biodiesel market; however, there is a Reform Initiative to the Law of Promotion and Development of Bioenergetics that looks for the obligatory introduction of biofuels to the Mexican market.
Biodiesel
Biodiesel is a mix of mono-alkyl esters of long fatty acid chains and is produced by the transesterification of vegetable oils or animal fats. The conventional catalysts are alkaline or acid (e.g., sodium hydroxide [NaOH], potassium hydroxide [KOH], sulfuric acid [H2SO4]) or prepared by grafting bases, salts, metal oxides, and enzymes on various supports such as alumina, silica, and resins. Currently, the transesterification through alkali catalyst is the preferred and most used method for biodiesel production at the commercial level due to economic reasons and high yields. It consists of the reaction of vegetable oils or animal fats with a short chain alcohol in the presence of NaOH or KOH [Equation (1)].3 The molar ratio is 1:3 (oil/alcohol) to obtain three fatty acid methyl esters (FAME) and glycerol as a by-product. Crude biodiesel (Figure 1) is produced from waste vegetable oil (upper fraction) and glycerol (bottom fraction).

Usually, the biodiesel is blended with diesel at different concentration levels. The most sold and used blends in the biofuel market are B2, B5, B10, and B20 because no important modifications are needed in the used systems. The capital letter “B” reflects the presence of biodiesel in the blend, and the following number denotes the percentage of the biofuel. Currently, new studies are being performed to assess the performance and properties of binary and ternary biofuel blends (e.g., biodiesel-diesel-bioethanol and biodiesel-diesel-biobutanol, among others).
Biodiesel oxidative stability is lower than diesel. The air, humidity, ultraviolet radiation, and microorganisms accelerate the biodiesel oxidation. The oxidation products of biodiesel are peroxides, hydroperoxides, mono-carboxylic acids (e.g., formic acid, acetic acid, propionic acid, and hexanoic acid), aldehydes, ketones, and alcohols. The presence of acids increases the total acid number and potential issues of corrosion.4-5
Among other features that make biodiesel more corrosive and with higher degradation potential than diesel are the following:6-7 biodiesel aging plays an important role—like vegetable oils it becomes rancid in a matter of weeks; it has higher electrical conductivity; it is more hygroscopic; and as a solvent may accelerate the degradation of polymers and elastomers, causing their swelling and loss of mechanical properties.
Therefore, the proper biodiesel handling from production, storage, and transportation to the end use must include proper materials selection and quality measures to ensure mitigation of corrosion failures and degradation issues along the different stages of the biodiesel supply chain.
Biodiesel Equipment and Materials
The biodiesel industry requires the utilization of corrosion- and degradation-resistant materials due to characteristics related to the chemical reagents and catalysts used in the biodiesel production process and to those of the fuel itself. The biodiesel supply chain starts in the feedstock production and logistics, followed by the biodiesel production, storage, and distribution. The final stage is the end use. Along the biodiesel supply chain, there is a wide diversity of systems, equipment, and components comprised of different metallic and polymeric materials. The biodiesel remains in contact with many of them, mainly in the production, storage, distribution, and end use stages (Figure 2).
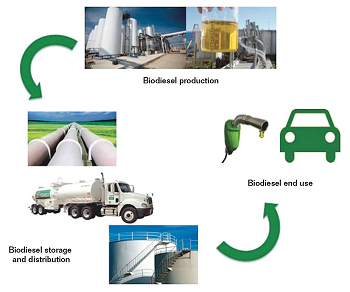
The most typical equipment, components, and their corresponding materials found in the biodiesel supply chain are summarized in Table 1.
Research has been done to assess the compatibility between biodiesel and metallic and polymeric materials. Table 2 shows the compatible and incompatible materials with biodiesel, taking into consideration the corrosion and degradation characteristics.
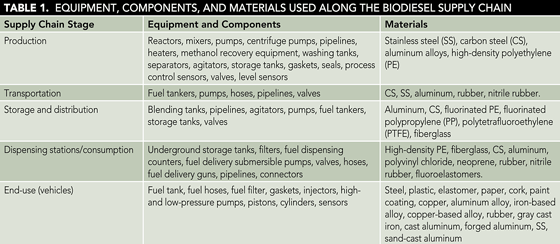
Materials Degradation
Pitting corrosion has been common in the metals that have been tested for their corrosiveness in biodiesel, such as aluminum, copper, copper alloys, and CS. SS was found to be immune to pitting corrosion in biodiesel applications and the most corrosion resistant.
Regarding polymers and elastomers, the most prone to degradation are those based on natural rubber materials. They are the least compatible materials with biodiesel since the biofuel behaves like a solvent. Biodiesel causes the swelling of polymers and elastomers and affects their mechanical properties. In the end, the material is hardened, and the brittleness is increased, leading to the point of rupture.9 Hereafter, the utilization of high-density PE and synthetic rubber such as fluoroelastomers is recommended because they have proven to be compatible with biodiesel.
Nowadays, there are many applications of biodiesel in different industries worldwide, as a fuel in the military, marine transportation, aircraft, public transportation, domestic vehicles, emergency power systems, furnaces, and boilers, among others. Biodiesel compatibility with different materials is an issue that requires special attention in every industry or sector where it is implemented. Hence, the biodiesel quality is ensured by producers and suppliers. An out-of-spec biodiesel could damage or compromise the proper operation of the systems, machines, or motor vehicles due to corrosion and degradation of materials, poor combustion, low performance, and filter clogging, among others.

The biodiesel must meet the requirements specified by the relevant American and European Union Authorities or the regulations of each country. Regarding corrosion testing of copper in the United States, the biofuels comply with the ASTM D130 “Test Method for Corrosiveness to Copper from Petroleum Products by Copper Strip Test.”10 A freshly polished copper strip is immersed in a specific volume of the fuel being tested and heated under conditions of temperature and time that are specific to the class of material being tested. At the end of the heating period, the copper strip is removed and washed, and the color and tarnish level are assessed against the ASTM D130 standard. The level of corrosion is evaluated as slight tarnish (1a, 1b), moderate tarnish (2a, 2b, 2c, 2d, 2e), dark tarnish (3a, 3b), and corrosion (4a, 4b, 4c). Normally, when copper corrosion is evaluated in biodiesel, the results obtained correspond to slight tarnish.
The Official Mexican Standard (NOM) NOM-EM-005-CRE-201511 is the official standard applied to petroleum products related to quality specs. It establishes all the requirements that the fuels must meet in each supply chain stage in the country. The fuels evaluated by the standard are gasoline, aviation fuel, automotive diesel, farm diesel, marine diesel, industrial diesel, heavy oil, aircraft gas, and liquefied petroleum gas. This NOM cites ASTM D130.
Copper was selected as an example to show the reactions that take place on its surface upon exposure to biodiesel:12
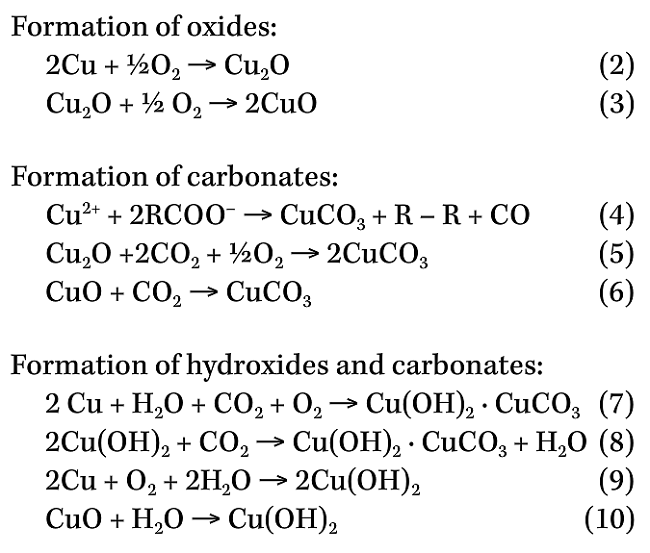
ASTM D130 is a qualitative test that only assesses the corrosiveness of copper exposed to a fresh sample of biodiesel. The main issue with this testing is the biodiesel aging and autoxidation nature that in a matter of weeks becomes more acidic and corrosive. Also, copper is not the only material that remains in contact with biodiesel in systems, equipment, and machinery; hence, a more comprehensive testing is performed to evaluate the corrosive characteristics of biodiesel in the presence of different materials through gravimetric, electrochemical, infrared spectroscopy, and scanning electron microscopy techniques. Other determinations applied to biodiesel and related with corrosion and degradation potential issues are the acid number, oxidation stability, and methanol content established in international standards.
Corrosion Prevention
The biodiesel industry applies corrosion inhibitors to retard the corrosion of equipment metallic parts.13 Research has been conducted on the inhibitory efficiency of amine-based corrosion inhibitors ethylenediamine, n-butylamine, and tertbutylamine against corrosion iron exposed to biodiesel. The amine-based inhibitors are absorbed on the surface of the metal and prevent corrosion by forming a barrier.14
Biodiesel has a low oxidative stability; therefore, the addition of antioxidants to biodiesel is a normal practice performed in the biodiesel industry to delay its autoxidation process and the formation of corrosive compounds.15 The antioxidants employed range from natural tocopherols to synthetics (e.g., butylated hydroxytoluene, tertiary butylhydroquinone, pyrogallol, propylgallate, and butylated hydroxyanisole). The synthetic antioxidants are widely used by the petroleum industry.
Conclusions
The production and consumption of biodiesel are being encouraged and expanded worldwide due to current efforts to reduce the dependency on fossil fuels and mitigate the problem of climate change. The biodiesel industry requires the utilization of corrosion- and degradation-resistant materials along the supply chain for equipment, machinery, vehicles, and systems such as SS, aluminum, fluoroelastomers, and high-density PE. The addition of corrosion inhibitors and antioxidants to biodiesel is also necessary to mitigate corrosion failures and degradation issues of metallic and polymeric materials.
† Trade name.
References
1 G. Knothe, J. Gerpen, J. Krahl, The Biodiesel Handbook (Urbana, IL: AOCS Press, 2005), p. 309, ISBN: 978-1-893997-79-0.
2 International Renewable Energy Agency (IRENA), “Global Bioenergy Supply and Demand Projections,” a working paper for REmap 2030 (2014), https://www.irena.org/publications/2014/Sep/Global-Bioenergy-Supply-and-Demand-Projections-A-working-paper-for-REmap-2030 (December 09, 2015).
3 G. Montero, et al., “An Overview of Biodiesel Production in Mexico, Biofuels—Status and Perspective,” Krzysztof Biernat, ed., https://www.intechopen.com/books/biofuels-status-and-perspective/an-overview-of-biodiesel-production-in-mexico (November 02, 2015), ISBN: 978-953-51-2177-0, InTech (2015), DOI: 10.5772/59400.
4 S. Schober, M. Mittelbach, “The Impact of Antioxidants on Biodiesel Oxidation Stability,” European J. of Lipid Science and Technology 106 (2004): pp. 382-389.
5 H. Tang, et al., “Quality Survey of Biodiesel Blends Sold at Retail Stations,” Fuel 87 (2008): pp. 2,951-2,955.
6 E. Torsner, “Solving Corrosion Problems in Biofuels Industry,” Corrosion Engineering, Science and Technology 45 (2010): pp. 42-48.
7 M. Coronado, et al., “Materials Technological Challenges for the Biodiesel Industry Development in Mexico,” in “Materials and Processes for Energy: Communicating Current Research and Technological Developments,” Formatex Research Center (2013), http://www.formatex.info/energymaterialsbook/book/279-288.pdf (November 24, 2015).
8 National Biodiesel Board (NBB), https://biodiesel.org/docs/ffs-performace_usage/materials-compatibility.pdf?sfvrsn=4 (January 03, 2016).
9 M. Coronado, et al., “Degradation of Nitrile Rubber Fuel Hose by Biodiesel Use,” Energy 68, 15 (2014): pp. 364-369.
10 ASTM D130-12. “Standard Test Method for Corrosiveness to Copper from Petroleum Products by Copper Strip Test” (West Conshohocken, PA: ASTM International, 2012).
11 NOM-EM-005-CRE-2015, “Norma Oficial Mexicana de Emergencia, “Especificaciones de Calidad de los Petrolíferos,” http://dof.gob.mx/nota_detalle.php?codigo=5413788&%20fecha=30/10/2015.
12 A. Fazal, A. Haseeb, H. Masjuki, “Corrosion Mechanism of Copper in Palm Biodiese,” Corros. Sci. 67 (2013): pp. 50-59.
13 N. Cheng, et al., Corrosion Inhibitors for Prolonged Protection of Military Equipment and Vehicles,” MP 55, 1 (2016): pp. 54-57.
14 B. Singh, J. Korstad, Y. Sharma, “A Critical Review on Corrosion of Compression Ignition (CI) Engine Parts by Biodiesel and Biodiesel Blends and its Inhibition,” Renewable and Sustainable Energy Reviews 16, 5 (2012): pp. 3,401-3,408.
15 J. Pullen, K. Saeed, “Experimental Study of the Factors Affecting the Oxidation Stability of Biodiesel FAME Fuels,” Fuel Processing Technology 125 (2014): pp. 223-235.