A wide variety of types of corrosion-inhibiting admixtures for reinforced concrete are known.1-3 These include organic corrosion inhibitors such as formulations based on amines and alkanolamines (amino alcohols). It has been demonstrated in various laboratory investigations4-9 that these products can prolong the time to corrosion initiation in chloride exposure environments, or that they can raise the critical chloride threshold, thereby decreasing corrosion rates. In recent literature reviews,1-3 however, it has been emphasized that the vast majority of results stem from short-term laboratory studies, and that long-term documentation on the effectiveness of organic corrosion-inhibiting admixtures under real exposure conditions is lacking.
Experimental Procedures
Specimens
In 1995, L-shaped concrete specimens were cast and installed along a highway in the Swiss Alps (Figure 1). During the winter season, the vertical wall elements are exposed to chloride-bearing splash water. These walls are 2.10 m in height, 1.70 m in width, and have a thickness of 200 mm. They are reinforced with two layers of carbon steel bars (12-mm diameter bars with 200-mm vertical and horizontal spacing). On the front side facing the highway, the cover depth is 15 mm; on the rear side, the cover depth is 30 mm. The front and rear reinforcing steel mats are electrically isolated from each other; however, cable connections to each mat from junction boxes mounted on the rear side of the elements permit measurements and electrical interconnection on the front and back layers of steel reinforcement. The electrical continuity between these two layers was established through the junction boxes to simulate realistic conditions, apart from the short interruptions that were occasionally needed for certain measurements.
Three types of concrete were cast (one L-shaped specimen per concrete type), but only the results of the reference concrete and the concrete containing the commercial corrosion-inhibiting admixture are reported. Both concretes contain equal amounts of a superplasticizer and an air-entraining agent (to avoid freezing damage). The cement type used was ordinary Portland cement (325 kg/m3) and the water/cement ratio was 0.45 to 0.46. The only difference between the two reported concrete types is that one of them contains 12 kg/m3 of the organic corrosion-inhibiting admixture, which is based on amino alcohols, and marketed by Sika as FerroGard901†.
Measurements
During the past 18 years of concrete specimen exposure, a number of periodic measurements were performed to document the field performance of the different concretes.10 These include:
• The galvanic current flowing between the front and back layers of the reinforcing steel. The interval for current measurement is three years.
• The potentials on the concrete surface facing the road.11 The potentials were measured with a saturated copper/copper sulfate (Cu/CuS04) reference electrode (CSE) positioned at different spots on the concrete surface, and mapped. The measuring grid was equally spaced in both horizontal and vertical directions (100 to 150 mm). The interval for potential mapping is three years.
• The chloride content in the exposed faces of the concretes. To determine chloride profiles, concrete powder was collected by drilling at intervals to a depth of 50 mm. The chloride content in the powder sample was analyzed by acid digestion and titration. The first chloride profile was taken after nine years of exposure, with one profile taken per wall element. At exposure times of 13, 16, and 18 years, two chloride profiles were taken per concrete wall element.
After 18 years of exposure, the concrete cover was removed from selected areas of the specimens with an electrically powered jackhammer to permit visual inspection of the corrosion state of the reinforcing steel.
Results and Discussion
Electrochemical Monitoring

Figure 2 shows the galvanic current measured between the front and back reinforcing steel mats in the wall elements over time. During the first seven years, these currents were below the measureable accuracy, thus negligibly low. In the reference concrete, the galvanic current started increasing at approximately eight to nine years of exposure, with a significant increase after ~16 years. On the other hand, no marked increase of the galvanic current was observed in the concrete with inhibitor during the entire 18-year duration of the field test. The increase in galvanic current measured in the reference concrete indicates the onset of corrosion on the front side of the wall element after approximately eight to nine years, while the negligibly low level of galvanic currents observed in the concrete containing the corrosion inhibitor suggests the absence of relevant macrocell corrosion. These results were supported by the potential maps of the wall elements.10
Visual Inspections
Over time, more cracks and rust stains appeared on the front surface of the reference concrete element than on the element made from the inhibitor-containing concrete. After 18 years of exposure, selected small areas of reinforcing steel of the front mats were visually inspected in both elements after locally removing the concrete cover. These inspected areas were selected based on the potential maps. In the reference concrete, parts of the reinforcing steel mats exhibiting relatively negative steel potentials (-350 mV vs. CSE) were found to have localized corrosion attack, with up to 1.5-mm deep corrosion pits. In contrast, the reinforcing steel in the concrete containing the admixed corrosion inhibitor was found to be essentially free from corrosion attack apart from a few small, superficial rust spots—even at the lower end of the wall element, which is the most severely exposed zone, with steel potentials of ~-250 mV vs. CSE.
Chloride Ingress
The chloride content data in the concrete, measured over time at different depths, were entered into a common model to describe chloride ingress into the concrete. This approach is based on Fick’s second law of diffusion and can be expressed as follows in Equation (1):12
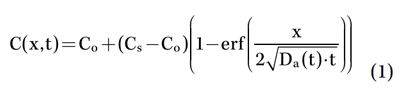
where C(x,t) is the chloride content at depth x and time t, C0 is the initial chloridecontent, and C8 is the so-called surface chloride content (all in % by mass of concrete. Da denotes the apparent diffusion coefficient (m2/s). To consider the time-dependency of transport processes through concrete, which changes due to proceeding cement hydration, carbonation, eventual formation of surface layers, etc., the apparent diffusion coefficient is usually expressed as a function of time, as shown in Equation (2):

where D0 denotes the diffusion coefficient at a reference age (e.g., 28 d), and α is the aging exponent. The model approach using Equations (1) and (2) is a strong simplification of reality.12 More complex models exist that may, under some conditions, allow more appropriate descriptions of chloride transport processes in concrete; however, these extended models require more input parameters, which are not available in the present work. Thus, application of the model according to Equations (1) and (2) in the present context is considered an appropriate approach that yields estimates of the evolution of future chloride content at a certain depth.
By assuming a negligible initial chloride content in the concrete (C0 = 0) and an aging exponent (α = 0.3 according to literature data12) for each point in time and for each sampled depth, the measured chloride content in the concrete was fitted to the model prediction (Equation [1]). The fitting parameters were D0 and Cs. The results are summarized in Table 1. The diffusion coefficients at reference time (28 d) are in the range of 2 to 3 x 10-12 m2/s. This is in agreement with literature results for concrete with Portland cement.12-13
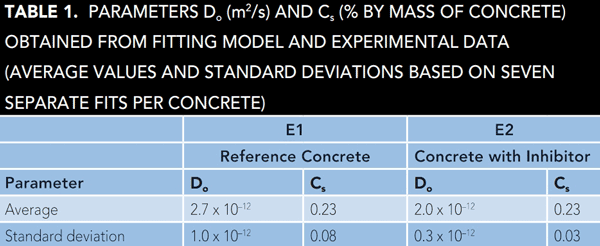
Table 1 indicates no dramatic differences in chloride ingress between the two tested concretes. Chloride ingress was only slightly slower in the inhibitor-containing concrete than in the reference concrete, with the apparent chloride diffusion coefficient D0 being ~30 to 40% higher in the reference concrete than in the inhibitor-containing concrete. Further indication of a slightly slower mass chloride transport in the presence of the inhibitor was obtained from electrical resistance measurements on embedded probes that suggested a higher concrete resistivity of the inhibitor-containing concrete than of the reference concrete, even after 18 years of exposure.10 In the literature, similar corrosion-inhibiting admixtures have occasionally been claimed to affect the transport properties, which was explained by the effect of internal hydrophobic coating or pore blocking.7,14
Projection of Time-to-Corrosion

The model describing chloride ingress permits extrapolating the results from the field test—obtained during 18 years of exposure—into the future. This is shown in Figures 3 and 4.
Based on results of both the nondestructive and destructive inspection techniques, the time of corrosion initiation, tini, can be estimated for the two concretes. In the reference concrete, corrosion initiated at approximately eight to nine years of exposure. In the concrete containing the corrosion-inhibiting admixture, 18 years of exposure may be considered a conservative estimate for the time to corrosion initiation.
The chloride content at the reinforcing steel mat’s cover depth (15 mm) at tini is defined as the critical chloride content Ccrit.15 For the reference concrete, Ccrit is estimated at 0.10% chloride by weight of concrete (~0.7% by weight of cement); and for the concrete with inhibitor, Ccrit is estimated at 0.11% chloride by weight of concrete (~0.8% by weight of cement). Thus, the difference in Ccrit for the concrete with and without the corrosion-inhibiting admixture is surprisingly small. The present results allow only a conservative estimate of Ccrit for the case of inhibitor-containing concrete, which is 10% higher than the reference concrete. Figure 4 shows that in order to achieve a chloride concentration that exceeds Ccrit, considerably longer exposure time would be required for the inhibited concrete to initiate corrosion than the reference concrete, and that delay is primarily associated with a reduction of the chloride diffusion rate. Having in mind that some tiny spots of corrosion were observed on the reinforcing steel in the inhibitor-containing concrete after 18 years, it is unlikely that tini will be much higher.
Figure 4 also shows a first-hand, estimated extrapolation of the present results for cover depths greater than the present 15 mm. For instance, tini becomes 40 years in the reference concrete and 80 years in the inhibitor-containing concrete with a cover depth of 25 mm. In short, tini is roughly doubled in the inhibitor-containing concrete. This beneficial effect is attributed to the combination of the influences on chloride transport properties and on Ccrit. While both effects are relatively small, their combination appears to be significantly prolong the service life in the presence of the corrosion-inhibiting admixture.
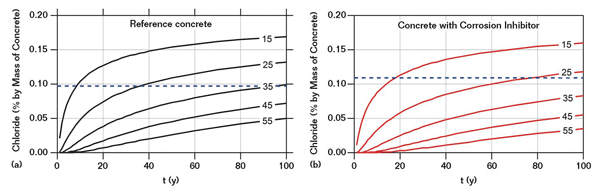
Both investigated concretes performed well. When extrapolated to realistic cover depths in the range of 35 to 55 mm, tini for the reference concrete is estimated to be close to or > 100 years (Figure 4). A likely explanation for this desirable behavior may be the special care that was taken during execution (casting, compaction, curing, etc.) and the resulting good-quality concrete. This highlights the importance of workmanship and careful execution on site for the durability performance of reinforced concrete structures.
Conclusions
In the present long-term field trial, the studied organic corrosion-inhibiting admixture (dosage 12 kg/m3) was sufficient to significantly delay corrosion initiation in conditions with exposure to chloride-containing splash water. While corrosion initiated at a cover depth of 15 mm after approximately eight to nine years of exposure in the reference concrete, the concrete with the corrosion-inhibiting admixture was, after 18 years, still essentially corrosion free.
A chloride ingress model was used to extrapolate the results after 18 years of exposure for both deeper cover depths and longer exposure times. As a rule of thumb, under the studied conditions, the inhibitor delayed the time to corrosion initiation by a factor of two, which illustrates the potential of this corrosion-inhibiting admixture to prolong the service life of reinforced concrete.
†Trade name.
References
1 C.L. Page, V.T. Ngala, M.M Page, “Corrosion Inhibitors in Concrete Repair Systems,” Magazine of Concrete Research 52 (2000): pp. 25-37.
2 B. Elsener, U. Angst, Corrosion Inhibitors for Reinforced Concrete (Cambridge, U.K.: Woodhead Publishing, 2016).
3 T.A. Söylev, M.G. Richardson, “Corrosion Inhibitors for Steel in Concrete: State-of-the-Art Report,” Construction and Building Materials 22 (2008): pp. 609-622.
4 B. Elsener, M. Büchler, F. Stalder, H. Böhni, “Migrating Corrosion Inhibitor Blend for Reinforced Concrete: Part 1—Prevention of Corrosion,” Corrosion 55 (1999): pp. 1,155-1,163.
5 H.E. Jamil, M.F. Montemor, R. Boulif, A. Shriri, M.G.S. Ferreira, “An Electrochemical and Analytical Approach to the Inhibition Mechanism of an Amino-Alcohol-Based Corrosion Inhibitor for Reinforced Concrete,” Electrochim. Acta 48 (2003): pp. 3,509-3,518.
6 H.E. Jamil, A. Shriri, R. Boulif, C. Bastos, M.F. Montemor, M.G.S. Ferreira, “Electrochemical Behaviour of Amino Alcohol-Based Inhibitors Used to Control Corrosion of Reinforcing Steel,” Electrochim. Acta 49 (2004): pp. 2,753-2,760.
7 M. Ormallese, M. Berra, F. Bolzoni, T. Pastore, “Corrosion Inhibitors for Chlorides Induced Corrosion in Reinforced Concrete Structures,” Cement and Concrete Research 36 (2006): pp. 536-547.
8 L. Benzina Mechmeche, L. Dhouibi, M.B. Ouezdou, E. Triki, F. Zucchi, “Investigation of the Early Effectiveness of an Amino-Alcohol Based Corrosion Inhibitor Using Simulated Pore Solutions and Mortar Specimens,” Cement and Concrete Composites 30 (2008): pp. 167-173.
9 L. Vyrides, E. Rakanta, T. Zafeiropoulou, G. Batis, “Efficiency of Amino Alcohols as Corrosion Inhibitors in Reinforced Concrete,” Open Journal of Civil Engineering 3 (2013): pp. 1-8.
10 U.M. Angst, M. Büchler, J. Schlumpf, B. Marazzani, “An Organic Corrosion-Inhibiting Admixture for Reinforced Concrete: 18 Years of Field Experience,” Materials and Structures 49,7 (2016): pp. 2,807-2,818.
11 ASTM C876-09, “Standard Test Method for Corrosion Potentials of Uncoated Reinforcing Steel in Concrete” (West Conshohocken, PA: ASTM, 2009).
12 L. Tang, L.O. Nilsson, P.A.M. Basheer, Resistance of Concrete to Chloride Ingress: Testing and Modelling (Abingdon, U.K.: Spon Press, 2012).
13 R.B. Polder, W.H.A. Peelen, “Characterisation of Chloride Transport and Reinforcement Corrosion in Concrete Under Cyclic Wetting and Drying by Electrical Resistivity,” Cement and Concrete Composites 24 (2002): pp. 427-435.
14 M.C. Brown, R.E. Weyers, M.M. Sprinkel, “Effects of Corrosion-Inhibiting Admixtures on Material Properties of Concrete,” ACI Materials J. 98 (2001): pp. 240-250.
15 U. Angst, B. Elsener, C.K. Larsen, Ø. Vennesland, “Critical Chloride Content in Reinforced Concrete—A Review,” Cement and Concrete Research 39 (2009): pp. 1,122-1,138.