Awareness of three parameters—safety, function, and service life—should be a part of every project. In order to address these parameters, consideration must be given to the design, choice of materials, construction methods, active and passive surface protection, maintenance, analysis of working conditions, and environmental factors.
In this article, incidents of corrosion damage involving personal injuries are identified, and the mechanisms to avoid or minimize them in the future are explained in detail. The origins of the failures are attributable to human error in the sense that the most elementary corrosion control procedures were overlooked.
“Corrosion Costs and Preventive Strategies in the United States,”1 a study published in March 2002, provided dramatic evidence of the impact of corrosion on every aspect of our society. This article offers case histories where unmitigated corrosion led to catastrophic situations with the loss of human lives. This was the consequence of improper design, poor choice of materials, and defective maintenance plans.
Small Bridge Collapse
In many countries, a network of canals or small streams is widespread, and thousands of small bridges were built to serve as culverts for water flow as well as supports for the roadways. Many of these small bridges are between 40 and 60 years old and were built according to a simple design patented by the ARMCO company in the United States. This design consists of a metal structure made of corrugated steel sheets bolted together to form an elliptical cross section or arch. This type of structure must necessarily be covered with compacted earth to share the road loads. At both ends of the metal arch (i.e., the entrance and exit of the channel), two concrete walls contain the road embankment and contribute to the support of the load. The metal sheets were hot-dip galvanized and coated with bitumen.
In 2011, the supporting archway at one of these bridges partially collapsed and was uniformly lowered, which resulted in a slight depression of the overlying paved road; however, this situation went unnoticed. In 2013, during a routine inspection, and following a warning from a citizen, a shallow subsidence of the roadway was observed.
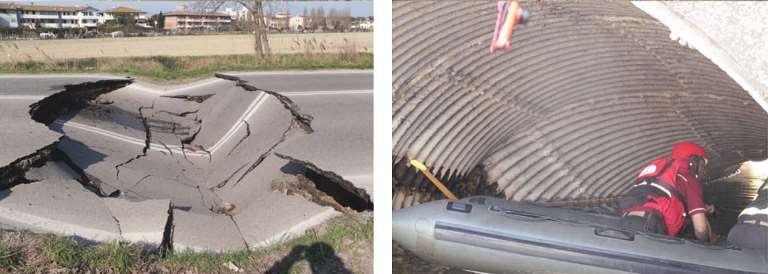
Only a few hours later the road collapsed as shown in Figure 1(a). Unfortunately, a car passed soon after the collapse, with deadly consequences for two persons. The origin of the failure, which was the collapse of the corrugated metal arch, is shown in Figure 1(b).
The bridge involved in the accident was built in 1975 and had performed its function of allowing the continuity of irrigation water flow, while supporting the road and its related traffic flow. Over the years, the road had undergone a continuous increase in traffic, and layers of both roadbed and asphalt were added. The collapse of the structure was the consequence of a set of causes, including the lack of maintenance, underestimation of the aggressiveness of the environment, uncontrolled increase of load, loose and scattered controls, and a very long operating time that approached its expected service life.
The tunnel environment was sheltered from the elements, open at the ends, and had a tendency to maintain a relative humidity higher than that of the surrounding environment. At the base of the tunnel, brackish water flowed with seasonally variable salinity levels that depended on the amount of inland fresh water and the marine water counter-flow from the nearby sea. On average, the brackish water had a pH of 7.5 and a chloride content between 4 and 18 g/L. Even a lower salinity content would be quite aggressive, since the conductivity would still be very high and the solubility of oxygen would be greater.
The bridge was considered to be oversized for the light traffic loads at the time of construction. No microbiologically influenced corrosion was detected during the investigation; however, heavy corrosion was caused by the microclimate in contact with the metal surface. Aggressive characteristics of the microclimate consisted of alternating partial immersion (wetting and drying zones) and adsorbed deposits of sediment containing moisture and chlorides.
An analysis of the collapsed sheets showed the extent of the corrosion. At the base of the arch, none of the original steel remained; it was replaced by a thick, compact layer of iron oxides, mostly magnetite. About 300 mm higher, the arch had been converted to loose corrosion products, completely eliminating any structural strength of the metal sheets.
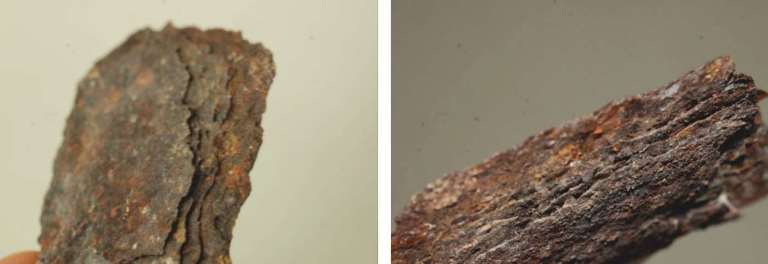
From Figure 2 it is possible to precisely match the environment with the corrosion pattern.2 The area 300 to 350 mm above the bottom of the arch was exposed to alternating wetting and drying (approximating a “splash zone”) and salt fog, and experienced the greatest corrosion rate. The presence of a galvanic couple with the magnetite formed at the base of the arch enhanced the dissolution rate. The splash zone acted as the anode, while the constantly submerged base of the arch behaved as the cathode. More compact, partially protective corrosion products formed on the base of the arch. Figures 3(a) and (b) compare corrosion products taken at 300 mm from the base with those collected at the base.
Chemical analyses using energy dispersive x-ray analysis (EDXA) were performed on the overlapping layers from the sample shown in Figure 3(b). These analyses showed the content of oxygen slightly increasing from the inside (steel substrate) toward the outside, very low amounts of chloride ions, and the presence of zinc concentrated near the residual metal. High quantities of silicon and calcium were near the surface, where sandy and calcareous concretions, partly leached over time, were still present. Corrosion was allowed to proceed to failure in the absence of an adequate maintenance program.
Crash Against a Jersey Barrier
A motor coach plunged off a viaduct, leaving 40 people dead in one of the worst road accidents in decades. The coach hit several vehicles before smashing through a barrier and toppling down a steep slope. The coach was taking ~50 people, including children, back home following a pilgrimage. The two main causes of the accident were:
a) The coach was old and had not been properly maintained. Its brakes failed. In order to avoid adjacent vehicles, the driver attempted to slow down by coasting along the guardrail and then the smoother concrete barrier (variously called a Jersey barrier, New Jersey barrier, or K-rail).
b) The barrier, officially tested as suitable to withstand a crash like the one caused by the brakeless coach, actually failed and was pulled free from its base for more than 100 m. Figure 4 shows the safe assembly of the barrier to the base, as well as its function in the event of a crash.
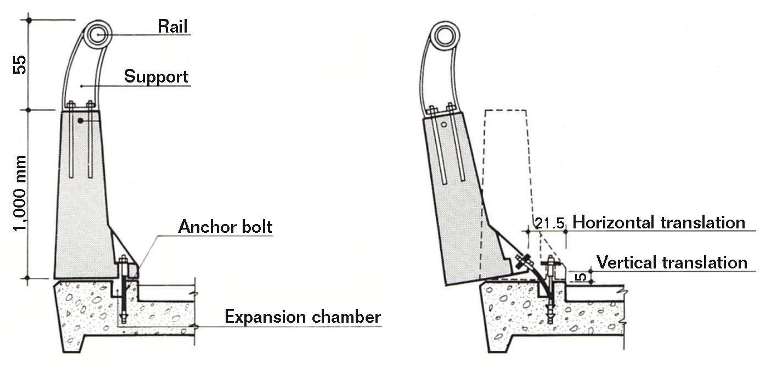
The driver trusted the suitability of the barrier to control the crash, but it failed. The anchor bolts at the base of the barrier were embedded in wells open to the environment. This was a critical design flaw. Water, deicing salts, dust deposits, and the absence of maintenance produced the effects shown in Figure 5.
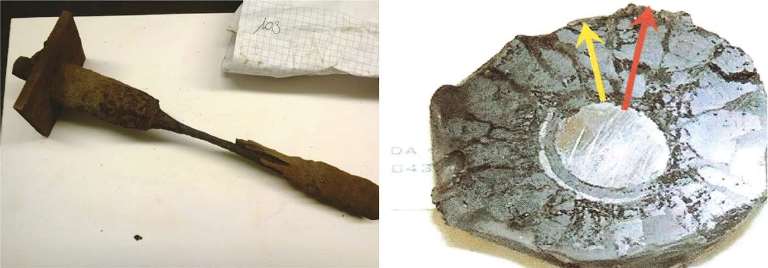
The photograph of the anchor bolt shows an advanced state of destruction by corrosion. The bolt is surrounded by a large layer of corrosion products consisting of iron oxides (magnetite and hematite) that developed over many years from progressive dehydration of the original rust. The final result of this process was a layer that acted as the cathode with respect to the original steel (magnetite is ~500 mV more noble than steel), which accelerated the corrosion process. At the same time, the layer of corrosion products surrounding the base of the bolt was partially protective because of the compactness and adhesion of those products. This is similar to the situation described for the small bridge plates. Analysis of this corrosion layer showed the presence of silicon, calcium, and chloride ions (this last element in minor amounts but in greater concentrations than the silicates that had leached from the cement).
Summary
A common feature in these corrosion failure examples is that basic corrosion control and proper maintenance were neglected. No mysterious, complex, never-previously encountered phenomena were involved. Fundamental awareness and involvement were lacking, which highlights the need for educating the individuals involved at all levels of a structure’s life, from cradle (designing) to grave (dismantling and disposing or recycling). It is necessary to disseminate the knowledge of even very simple corrosion principles to avoid the most elementary mistakes and reach the primary objectives of corrosion control: a long and safe service life. Unfortunately, awareness of the risk of corrosion is not yet universally understood, so human lives are still in danger because of avoidable corrosion catastrophes.
References
1 G.H. Koch, et al., “Corrosion Costs and Preventive Strategies in the United States,” Federal Highway Administration, FHWA-RD-01-156, March 2002.
2 R.W. Revie, ed., Uhlig’s Corrosion Handbook, 3rd ed. (Hoboken, NJ: John Wiley & Sons, Inc., 2011).