A team of researchers at Penn State University (University Park, Pennsylvania, USA) are investigating how contaminants in power plant water cycles affect the integrity of steel pipes and tubing in power generation systems.
The team is being led by Derek Hall, an energy engineering researcher in Penn State’s John and Willie Leone Family Department of Energy and Mineral Engineering. Hall’s team includes Akash Ganesan, one of Hall’s doctoral students, and Serguei Lvov, a professor of energy and mineral engineering and materials science and engineering.
Establishing Project Objectives
In collaboration with Penn State’s Institutes of Energy and the Environment’s Center for Quantitative Imagining, the team is looking to expand their understanding of corrosion processes in extreme environments. The objective of the project, sponsored by the U.S. Electric Power Research Institute (EPRI), is to provide evidence of how different water contamination levels and types change the extent of damage observed from corrosion processes in a laboratory setting.
“The guidelines are designed to help power plants manage chemistry process-related issues to minimize risk of corrosion-related features,” says Brad Burns, area manager of process operations and chemistry for EPRI. “The key factors are time and concentration of corrosive contaminants. Penn State has the capability to simulate boiler water conditions with temperature, pressure, and water chemistry. Our goal is to learn about when active corrosion initiates through use of both electrochemical methods and the nano-computed topography scans.”
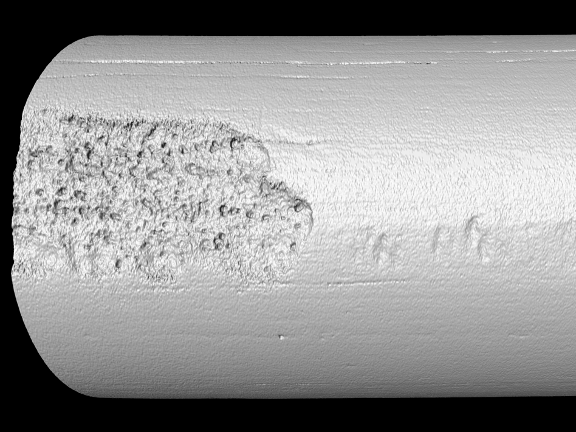
Limits of Historical Means
However, corrosion risks are notoriously difficult to study, according to Hall. “Normally, we need to destroy samples during the process of detecting any localized corrosion or corrosion damage under the naturally forming surface oxide layers,” he says. “In this study, we are using nano-computed tomography to nondestructively monitor how base metal and corrosion product layers change with extended exposures to corrosive fluids.”
Hall said that this work helps power generation systems—such as coal, nuclear, geothermal, and natural gas-fired plants—understand how to minimize corrosion risks while operating their systems in concert with renewable energy systems. Some sources of power, like solar and wind, are intermittent by nature and require other sources to adjust their electrical power outlets to stabilize the grid.
“Water cycle power plant systems are designed to run at an optimal power output and operate less efficiently when changes occur,” Hall says. “Now, they are being asked to shift their power output much more frequently, so operators need to manage corrosion risk in more dynamic operating conditions.”
As more renewable sources are added, the frequency of power shifting and cycling increases, says Hall. “Many systems reliant on water cycles struggle to adjust quickly,” he says. “The current energy transition is putting considerable pressures on how power plant operators manage their systems.”
Burns says that project outcomes will help with reliability and availability of the assets to produce power by creating guidelines that will help manage risk to ensure this can be done over the long term.
“Industry guidelines set limits on how long you can operate with certain levels of contaminants within your water cycle,” Hall says. “However, these limits are based on industry experiences, which vary considerably. Some plants may see severe damage in short amounts of time, whereas others observe minimal damage under the same conditions. We aim to provide experimental data to better pinpoint the causes of damage due to high contamination levels to better define these limits and the implications of operating beyond them.”
Benefits of New Technologies
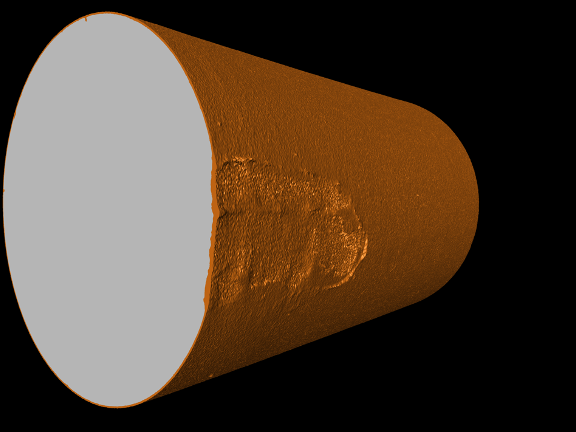
Burns says Penn State offers key technologies that help EPRI achieve their goal. “We also gain tremendous benefit from collaborating with Derek and his team,” he says.
The monitoring is being completed with a piece of equipment called the Zeiss Versa 30. This nondestructive system provides three-dimensional imaging of biotic and abiotic materials. The Zeiss Versa is part of the Institutes of Energy and the Environment’s core facilities, specifically the Center for Quantitative Imaging (CQI).
“The Zeiss team has been instrumental in helping us use X-ray computed tomography to quantify corrosion rates associated with our simulated exposure tests,” Hall says. “The instrument allowed us to nondestructively track how the base metal and oxide layer changed with increasing exposure time and contamination levels.”
According to Sara Mueller, CQI’s research technologist who is running project samples on the Zeiss, the Penn State project demonstrates the power of computed tomography technologies. She says that divergent materials must absorb different amounts of X-ray. The difference in photon counts detected by the camera allows researchers to see different density materials as part of the same sample, in this case oxides and base metals.
“The Zeiss allows researchers to ask, ‘what’s inside?’ at a resolution that was previously only visible by cutting a sample and using other technology, such as a scanning electron microscope,” says Mueller. “Cutting a sample introduces its own variation that we do not have in computed tomography. Additionally, we can track the same sample over time and across treatments as the researchers did in this study.”
Mueller adds that beyond metals, CQI can measure small animal brain regions, embryonic bone, individual fibers, pores, and cracks that would otherwise be invisible. “With the right size and density of sample, we can achieve a resolution of about 300 nanometers, which allows us to being to visualize micron-sized features,” she says.
“To put this in perspective, that’s examining features less than 1% of the width of human hair,” she concludes. “We are discovering features we didn’t even realize to ask questions about. It’s really exciting.”
Source: Penn State, www.psu.edu.
This article was originally published in the December 2022 edition of Materials Performance. Republished with permission.