Deicers are liquid or solid chemicals that are used for winter maintenance of roadways. The deicers used by winter maintenance agencies are mainly made of chloride-based salts as a freezing point depressant, which can be sodium chloride (NaCl), potassium chloride (KCl), calcium chloride (CaCl2), and magnesium chloride (MgCl2).1 NaCl is the most common deicer in the United States due to its availability and low cost; however, at temperatures below -12 °C (10 °F), NaCl becomes ineffective and other salts are used, instead.2
Different parts of vehicles are vulnerable to corrosion damage caused by deicers.3 These parts can be hydraulic, electrical, and structural components of the vehicles.4 A study in a Scandinavian country found that cars driven on salt-free roads were 50% less vulnerable to cosmetic corrosion than cars exposed to road salt.5 One study has estimated that vehicular corrosion cost due to road salts is at least $113 per 0.9 ton.6
In a more recent study by North American agencies, the total cost of current corrosion management and corrosion risks related to deicer exposure was estimated to average $1 million to $14 million per year.7 Figure 1 shows the corrosion damage caused by road salts on different truck components.

This article aims to provide an in-depth understanding of failure in a truck component caused by deicer corrosion. In this regard, a pitted exhaust pipe clamp (EPC) of a truck was assessed by electron probe microanalysis (EPMA). The deicer responsible for the corrosion also was analyzed by liquid chromatography mass spectroscopy (LC-MS), Fourier transform infrared (FTIR) spectroscopy, x-ray fluorescence (XRF) spectroscopy, total reflection x-ray fluorescence (TXRF) analysis, energy-dispersive x-ray spectroscopy combined with electron backscatter diffraction (EDS-EBSD), and chloride sensor.
Experimental Procedure
Based on the composition reported by the Butte Department of Transportation, the deicer used in the Butte area consists of NaCl, MgCl2, CaCl2, KCl, and beet extract solution (the concentration of each component was not reported).
The functional groups of the deicer were obtained using the FT-IR spectroscopy technique via a Thermo Scientific Nicolet iS 10† spectrometer. The IR spectra was collected over the wave number range of 3,900 to 400 cm−1.
The LC-MS technique was used for in-depth analysis of the chemical composition of the deicer. LC-MS was conducted by a Synapt G2-S mass spectrometer† in positive ion mode.
A ThermoARL Advant’XP+† sequential x-ray fluorescence spectrometer was used for XRF analysis. Chlorine can be variably lost during the fusion process, so its data should be considered semi-quantitative. Therefore, Cl data was not considered in the results.
An S4 TSTAR† spectrometer was used for TXRF analysis of the deicer sample. This method of surface element analysis is regularly used to analyze trace element values. Since TXRF is not able to detect sodium (Na), an EDS-EBSD system was used to determine the amount of Na in the deicer. Chloride concentration in the deicer sample was determined using a chloride sensor.
Results and Discussion
FT-IR Spectroscopy
Figure 2(a) presents the FT-IR spectrum of the deicer. The bands at 3,405 and 3,246 cm-1 are attributed to the O-H stretching of alcohols and N-H stretching of amides. The absorption bands at 2,250 and 1,801 cm-1 could be assigned to ‒C≡C‒ stretching of alkynes and C=O stretching of carbonyls, respectively.
Two peaks at 1,635 and 1,616 cm-1 could be attributed to N-H bending of primary amines. Multiple bands in the range of 1,508 to 1,419 could be associated with C=C stretching of aromatics. The peaks at 1,107 and 1,035 cm-1 are related to C-N stretching of aliphatic amines. Finally, the band at 611 cm-1 could be due to C-Cl stretching of alkyl halides.
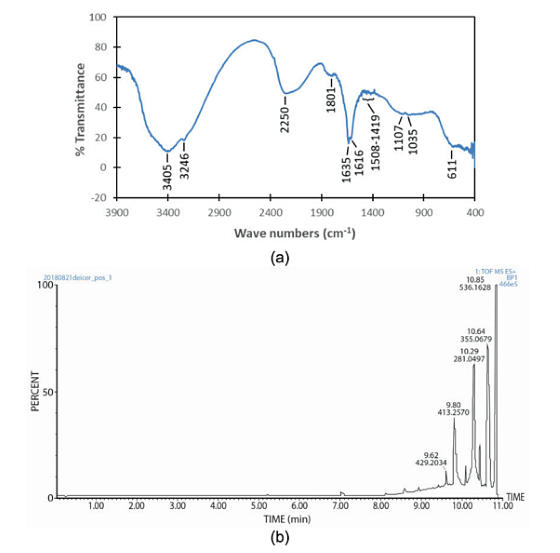
Liquid Chromatography—Mass Spectroscopy
LC-MS data for the deicer are presented in Figure 2(b) and Table 1. According to the LC-MS results, the deicer contained C, H, N, O, P, S, Mg, Na, and Cl. It shows that this deicer contains organic compounds. This agrees with FT-IR results and the composition reported by the Butte Department of Transportation.
It is noteworthy that the organic compounds that contain N, P, and S have a polar function. These compounds can adsorb on the metallic surface and protect it from corrosion.
Table 2 presents the chemical composition of the mixture of sand and debris found along the roadside after winter maintenance operations. This mixture is made of 71.94% SiO2 and other minerals such as MgO, CaO, Na2O, and K2O. The chemical composition of this mixture is similar to beach sand that is exposed to seawater.⁹ On the other hand, the river sand that has experienced freshwater has more than 95% SiO2 with no Mg, Ca, Na, and K ions.9
Seawater is a chloride-based solution similar to deicing chemicals. Therefore, it can be concluded that deicing chemicals are present in the debris found along the roadside.
Table 3 shows the deicer chemical composition obtained using a combination of TXRF and EDS-EBSD and chloride sensor. According to this table, the deicer is made of Mg, S, Cl, K, Ca, V, and Na. The results are consistent with previous analyses and indicate the presence of chloride-based salts (mainly MgCl2) and organic matter in the composition of deicer.
To study the chemical composition distribution around a typical corrosion pit on the EPC part, EPMA analysis was employed, as shown in Figure 3. This technique enables mapping of different elements in a defined surface area. The overall patterns show increasing Fe, O, and Cl concentration around the rim of the pit. No increase in the concentration of Mg on the edge of the pit was observed, which suggests that Mg does not have any direct role in deicer corrosion. It may have an indirect role by absorbing water in the dry hours and providing a suitable environment for the corrosion process.
The increase in the concentration of Fe, O, and Cl indicates the formation of iron chloride and iron oxide(s) according to the following reactions known to be underlying chloride-induced corrosion of steel.
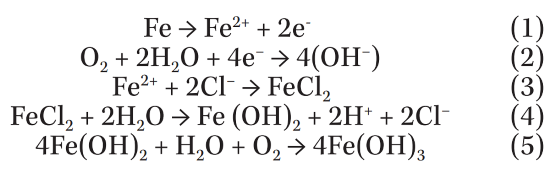
These reactions are proposed for pitting of iron in chloride-containing solutions at near-neutral pH range.10-11 They clearly show that the Cl ion has an important role in developing the pitting damage. Chloride ion breaks down the coating and provides a discontinuity in the protective layer, which becomes a suitable spot for initiation and growth of pits.
The pitting propagation would be due to the difference between electrical charges of the inside and outside of the pit because of anodic reactions inside of the pit and cathodic reactions around the pit. This causes a localized pH gradient and, as a result, the propagation of pitting.
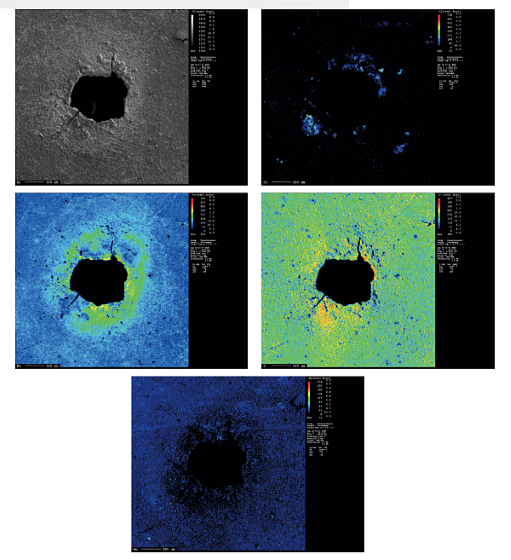
Conclusions
This work reports a case study on the corrosion of a truck due to roadway deicer in Butte, Montana. The chemical composition of the deicer was determined by LC-MS, FT-IR, XRF, TXRF, EDS-EBSD, and chloride sensor. Corrosion was studied by surface analysis and the following conclusions were obtained:
- According to FT-IR analysis, the deicer consisted of the functional groups of alcohols, amides, primary and aliphatic amines, alkynes, carbonyls, aromatics, and alkyl halides.
- Based on the analyses, the deicer is made of chloride-based salts (mainly MgCl2) and organic matter.
- Pitting corrosion was observed in the truck part exposed to the deicer.
- An increase in the concentration of Fe, O, and Cl was observed in the rim of the pit, which can be due to the formation of iron oxide(s) and iron chloride constituents.
- Mg cations did not exhibit any direct effect on the deicer corrosion.
- Corrosion of the truck part was attributed to the chloride anions in the deicer.
Acknowledgments
The authors would like to acknowledge the Butte Department of Transportation for providing information on the chemical components of deicer and deicer sample, and Bill Field Trucking, LLC for access to their trucks, steel sample, and mixture of deicer and sand.
† Trade name.