Corrosion of steel rebar is a significant cause of concrete degradation. The build-up of corrosion products on the rebar surface exerts pressure on the concrete, which leads to cracking and spalling of the concrete cover. The decrease in pH of a concrete structure with time due to carbonation increases the susceptibility of the rebar to corrosion.
Exposure to chlorides, through atmospheric deposition near seacoasts or through the use of road deicing salts during winter maintenance periods, will also accelerate deterioration of reinforced concrete structures. If left unchecked, rebar corrosion results in expensive repairs, decreased load carrying capacity, and premature structure replacements.
Steel may be protected against corrosion by connecting it to a zinc anode. When two different metals are in electrical contact, and bridged by an electrolyte, current flows from the anodic or base metal to the cathodic or more noble metal. In the case of iron (steel) and zinc, the base zinc anode protects the more noble iron. This is the basis of cathodic protection (CP).1
Impressed current CP (ICCP) systems consist of anodes that are connected to a power source that provides a continuous direct current. A control system is needed to provide enough current for the ICCP system to protect the target structure. CP transformer-rectifier units automatically adjust the operating voltage to maintain the optimum current output. For large complex target structures, such as bridges, the ICCP systems are often designed with multiple independent zones.2
The Oregon Department of Transportation (ODOT) selected thermal sprayed zinc coatings for use as anodes in ICCP systems on the historic Cape Creek, Yaquina Bay, and Depoe Bay bridges.3 These three bridges are magnificent arch bridges on U.S. Route 101 designed in the Art Moderne style and listed in the National Register of Historic Places. The decision to repair these older bridges with a zinc anode-based ICCP system involved issues of public finance and safety, but also historical significance. The sprayed zinc anodes complement the historic structures, all while protecting the rebar by reducing the rate of corrosion, regenerating an alkaline environment around the rebar, and moving chloride ions away from the rebar.
Thermal Sprayed Zinc Anodes
Thermal sprayed zinc anodes are well established as a component of ICCP systems for the corrosion protection of reinforced concrete structures.4 The arc spray process, which propels molten zinc metal droplets onto the concrete surface,5 was selected by ODOT since it could be easily applied to the complex shapes found on the three bridges. Zinc anodes minimize the dead load added to the structure, and the gray color of zinc also looks very much like concrete, which was an important consideration for the rehabilitation of the historic bridges. Also, the low electrical resistivity of zinc allows uniform distribution of the CP current.6
The zinc anode is produced by impacting semi-solid droplets of zinc on a roughened concrete surface. This produces a metallic zinc layer with micro-pores and oxide coatings on the individually solidified particles. Coating thickness is dependent on the time of spray, and the anodes were a minimum 500 µm (20 mils) thick on the three bridges.7 Porosity typically makes up 5 to 7% of the coating volume. The overall coating has characteristics like solid metallic zinc.
ODOT practice for installation of thermal sprayed zinc anodes includes initial abrasive blasting of the entire surface in the work area on the bridge, followed by any needed repair of the concrete and the installation of the anode terminal plates. Then the concrete surface receiving the zinc anodes is grit blasted before thermally spraying the zinc anodes onto the concrete surface.8
The ICCP zinc anode zones are each ~300 to 500 m2 in size. Each zone has a connection to the reinforcing steel (cathode) and the thermal sprayed zinc (anode). The cathode connections are placed near the center of the zone and spaced ~3 m apart. For redundancy, at least two anode connection terminal plates are installed for each zone. At this location, the ICCP system positive wire terminates and the electric current is distributed to the zinc anode. The ICCP systems were operated by ODOT with a target current density (CD) of ~2.2 mA/m2, which was found to adequately protect the steel reinforcement over a wide range of conditions.
The sound or rehabilitated concrete surface receiving the zinc anodes is blasted using a nonmetallic grit to provide an ICRI CSP 4 surface profile. It was found that abrasive blasting with a hard and dense grit material using a lower air pressure provided the best adhesion. However, it was important not to expose too much of the aggregate phase.9
The adhesion of the zinc anode to the concrete surface is mainly governed by the mechanical interaction of molten zinc droplets with the surface. The depth profile, as measured by the root mean square roughness, is the main parameter that can be related to the bond strength of the thermal sprayed zinc anode.10 The adhesion of thermal sprayed zinc on concrete does not appear to be adversely influenced by severe freeze-thaw cycling.11
Cape Creek Bridge
The first ODOT project to use thermal sprayed zinc anodes for ICCP was the Cape Creek bridge. The zones were identified and selected based on architectural features, to have similar current requirements. In total, the sprayed surface area was 9,500 m2, with a coating thickness of 500 µm. It was initially required to have the concrete surface at 21 °C or warmer to keep it dry, and then supplemental surface heating applied immediately prior to zinc application to bring the concrete surface temperature to ~120 °C.12 ODOT has since shown that preheating is of no practical value unless the concrete is wet.13 The bridge rehabilitation took 21 months and was completed in December 1991. The ICCP system has operated since January 1992.
Yaquina Bay Bridge
The Yaquina Bay bridge has the largest application of thermal sprayed zinc anode surface area of the three bridges discussed here. Between August 1992 and February 1994 (19 months), the arch spans were rehabilitated and the ICCP system was installed. This phase involved the thermal spraying of over 18,000 m2 of zinc anode. After the arches, it took 10 months to remediate the concrete and install the thermal spray zinc anodes on the south approach spans between September 1996 and June 1997. In this phase, an additional 6,000 m2 of zinc anodes were sprayed on the bridge.
Uneven consumption of the zinc anodes was observed on some areas of the Yaquina Bay bridge arches and southern spans. Investigations revealed that the rectifier voltage and currents were up to 10 times higher than the set points. The cause was due to the excessive current output from faulty rectifier controllers, and possibly from the application of humectants being tested in these areas to improve the electrical characteristics of the anodes.14 Anode geometry, interference from other electrical systems in the area, and different local environmental conditions, such as time of wetness, may also contribute to uneven CD.14
Depoe Bay Bridge
The installation of the ICCP system on the Depoe Bay bridge (Figure 1) took 32 months from December 1993 to August 1996 and involved the thermal spraying of 5,900 m2 of zinc anodes. Of the three bridges, the Depoe Bay bridge has the smallest anode area; however, significant concrete repair was required. The bridge is also located in a sensitive area that includes a marina and the Depoe Bay Wayside Ocean state park. It was important that the concrete remediation and zinc thermal spray work was performed with minimal social or environmental impact.
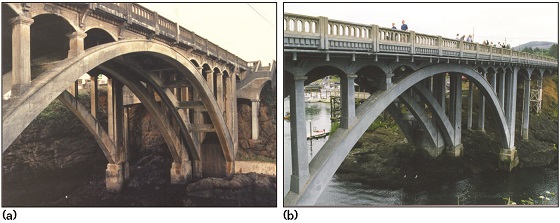
Economics
The projects can be broken down into five broad components: mobilization and traffic, bridge rehabilitation, concrete preparation and repair, enclosure, and cathodic protection.15 The largest cost component of the rehabilitation costs for the three bridges was the enclosure for protecting workers on site from the weather and for capturing any possible emissions. The enclosure also provides climate control for the thermal spray application, including adequately low humidity, surface temperature high enough to ensure dry concrete surfaces, ambient temperature, and minimal dust escaping.
The enclosure cost was influenced by operating in sensitive areas, and the high level of protection chosen by ODOT. The enclosure costs on the Yaquina Bay and Depoe Bay bridges were double the cost of the Cape Creek bridge because significantly more concrete repair work was needed and more of the bridge span is over open water. The average ICCP system, including anode installation, was 27% of the total cost of the bridge rehabilitation. The anode installation itself was only 14% of the total cost. By using the zinc-based ICCP system, ODOT saved the three bridges at a fraction of the replacement costs. The rehabilitation of the three bridges cost ~35% of the estimated replacement costs for new bridges.7
Monitoring costs for the Cape Creek Bridge ICCP system have been $1,200 per year for site visits and data acquisition. The power requirements to operate the ICCP systems are small. For example, the ICCP system of the Cape Creek Bridge requires ~15,000 kWh per year (which is equivalent to a cost of $700 per year). These costs are very small compared to overall bridge maintenance costs.15
The zinc anode-based ICCP systems have been working well to protect the iconic reinforced concrete bridges, meeting the predicted anode life. Based on this performance, ODOT awarded a new contract in 2018 to replace the oldest anode installation and reinstall a new zinc anode-based ICCP system on the Cape Creek bridge, 27 years after the initial installation.
Conclusions
ODOT has successfully extended the lives of three iconic bridges by using thermal sprayed zinc coatings for use as anodes in ICCP systems on the historic Cape Creek Bridge, the Yaquina Bay Bridge, and the Depoe Bay Bridge. The arc sprayed zinc anodes were easily applied to the complex shapes found on the bridges. Zinc anodes minimize the dead load added to the structure, and the gray color of zinc was an important consideration for the rehabilitation of the historic bridges. Also, the low electrical resistivity of zinc allows uniform distribution of the CP current.
The 25-plus-year experience at ODOT shows that the service life of reinforced concrete structures can be economically extended by using metallic zinc anodes to protect the steel reinforcement from further corrosion.
References
1 F. Porter, Zinc Handbook (New York, NY: Marcel Dekker, Inc., 1991).
2 W. Von Baeckmann, et al., eds. Handbook of Cathodic Corrosion Protection, 3rd ed. (Houston, TX: Elsevier, 1997).
3 S. Bullard, et al., “Thermal-Sprayed Zinc Anodes for Cathodic Protection of Steel-Reinforced Concrete Bridges,” InterCorr’96, DOE/ARC-1996-004 (Houston, TX: U.S. DOE, 1996).
4 R.G. Powers, A.A. Sagues, T. Murase, “Sprayed Zinc Galvanic Anodes for the Cathodic Protection of Reinforcing Steel in Concrete,” Materials: Performance and Prevention of Deficiencies and Failures, held August 10-12, 1992 (New York, NY: ASCE, 1992).
5 F. Prenger, “Thermal Spraying with Zinc and Zinc Alloys for Offshore Wind Energy Plants,” Proc. Windforce 2014, held June 17-19, 2014 (Bremen, Germany: Windenergie Agentur, 2014).
6 G. McGill, T. Shike, “Rehabilitation and Preservation of Oregon’s Historic Concrete Coastal Bridges,” Transportation Research Record 1601 (1997).
7 B.S. Covino, et al., “Performance of Zinc Anodes for Cathodic Protection for Reinforced Concrete Bridges,” Federal Highway Administration, FHWA-OR-RD-02-10, March 2002.
8 “Prepare Surfaces and Apply Zinc Anodes,” ODOT 2018 Boilerplate Special Provisions, SP01260, 2018.
9 R. Brousseau, M. Arnott, S. Dallaire, “The Adhesion of Metallized Zinc Coatings on Concrete,” CORROSION/93, paper no. 331 (Houston, TX: NACE International, 1993).
10 J.G. Legoux, S. Dallaire, “Adhesion Mechanisms of Arc-Sprayed Zinc on Concrete,” J. of Thermal Spray Technology 4, 4 (1995).
11 R. Brousseau, M. Arnott, B. Baldock, “LongTerm Factors Influencing the Adhesion of Metallized Zinc to Concrete,” J. of Thermal Spray Technology 5 (1996): pp. 49-52.
12 G.R. Holcomb, et al., “Electrochemical Aging of Thermal-Sprayed Zinc Anodes on Concrete,” Thermal Spray 1996: Practical Solutions for Engineering Problems, Proceedings of the 9th National Thermal Spray Conference (Metals Park, OH: ASM International, 1996), pp. 185-192.
13 G.R. Holcomb, et al., “Thermal Spray Coatings for Coastal Infrastructure,” Tri-Services Corrosion Conference (Wrightsville Beach, NC: U.S. DOE, 1997).
14 J.S. Tinnea, Cryer C.B., “Corrosion Control of Pacific Coast Reinforced Concrete Structures: A Summary of 25 Years Experience,” Proceeding of First International Conference on Applications Heritage and Constructions in Coastal and Marine Environment (Lisbon, Portugal: MEDACHS, 2008).
15 G.R. Holcomb, C.B. Cryer, “Cost of Impressed Current Cathodic Protection for Coastal Oregon Bridges,” MP 37, 7 (1998).