The application of surface treatments— coating layers with properties superior to those of the substrate—generally are used to improve the service life of equipment in industrial settings.1-2 Controlling the surface layer’s mechanical properties is considered to be an important attribute for current engineering applications. Minor damage of the surface sometimes results in mechanical component failure, which has caused fatal accidents. Surface treatments can also provide a longer service life with a consequent reduction of total costs.3
Tungsten carbide-based (WC-based) composite layer coatings have good resistance to abrasive and erosive wear due to the presence of WC particles that are dispersed in an alloy binder such as nickel or cobalt.4 Thermal spraying, such as high-velocity oxygen flame spraying, plasma spraying, wire arc spraying, flame spraying, and other techniques,5 is a useful technology to produce WC-based composite layer coatings. Because it is a mechanically bonded coating, however, thermal spraying has low bond strength between the coating layer and the substrate. Also, it exposes the WC powder to oxygen or water vapor, which leads to porosity and oxide formation.3
Another process for preparing a WC composite layer coating is based on infiltration brazing technology. In brazing, the substrate is wetted by the melted filler metal and a metallurgical bond is formed. The bond strength is superior to that of thermal sprayed coatings.6 It can be applied to curved, inner, or outer surfaces simultaneously, which can reduce the cost of machining the coating surface after brazing. It also has less distortion, lower residual stresses, and lower substrate dissolution.7
WC dispersed in nickel (WC-Ni) is a cermet, which is a metallic matrix with dispersed ceramic particles, that is widely used in industries due to its high hardness and good wear resistance. Gao, et al.8 reported on a WC-Ni brazed coating made with WC- and Ni-based metal powders applied through high-temperature vacuum brazing. Lu and Kwon6 reported that wear resistance of the WC-Ni brazed coating is better than other overlaid coatings under wet sand abrasive wear and rotating slurry erosive wear. Klaasen, et al.9 investigated the strength and residual stress between a WC-Ni brazed coating and the substrate. They found that the plasticity and proof stress of the filler metal affect the reliability of WC-Ni brazed coating.
The extremely high resistance of the WC-Ni brazing layer to wear and corrosion is effective for many applications, such as coal slurry transportation, seawater cooling systems, flue gas desulfurization (FGD), and especially power plant tubes, where the combined erosion and corrosion phenomenon widely occurs. For example, rotating impellors used in coal slurry transportation, seawater cooling systems, and FGD often suffer attack from solid particles and corrosion, as shown in Figure 1.
In many engineering systems, this is a significant degradation mechanism. It involves mechanical wear damage caused by the erosion generated by solid particles and surface material loss from exposure to a corrosive medium. Erosion and corrosion may create a synergistic effect that produces a wear rate that is greater than the sum of each process alone.10-11
In aggressive environments, the discrete understanding of corrosion resistance or mechanical erosion resistance is not sufficient to select an effective method of material protection from the combined attack of erosion and corrosion.
Coating techniques could provide a solution for protecting the surface, but it is very difficult to uniformly coat an impellor due to its complex geometry. The vacuum brazing technique, however, can apply a uniform coating on an impeller using a rotation system in the vacuum furnace.
Until recently, limited information on the combined erosion and corrosion performance of WC coating materials was available. M. Reyes and A. Neville12 investigated the degradation mechanism of WC-based cermets for drilling tools. They reported that erosion models have limitations for liquid-solid impingement conditions because of corrosion and complex particle trajectories. E.J. Wentzel and C. Allen10-11 noted the effect of different binders on the resistance of WC coatings to combined erosion and corrosion. They also observed that binder removal is the main degradation mechanism during slurry erosion.
In addition, A. Neville, et al.,13 investigated the behavior of WC-based metal matrix composites in liquid-solid slurries with different erodent sizes and loading. They reported that the erodent size is a very important parameter that affects the total mass loss of materials. However, little research work has been done on the combined erosion and corrosion performance of WC-Ni brazed coating materials. For this article, an improved manufacturing process for rotary brazing was studied with simulation work to find suitable process conditions.
Materials
The coating powder mixture was made using WC powders with the grade size of 1 μm and a Ni–Cr–Si brazing alloy powder with the grade size of 5 μm. The chemical composition is 8 wt% Cr, 4.5 wt% Si, 3 wt% B, 3 wt% Fe, and the balance Ni. Organic binders were added with the materials by ball milling, and then the mixture was rolled into a flexible sheet. Polymethylmethacrylate was used for the organic binder and was agglomerated into carbide particles and removed by sintering. The organic binder provided good formability to the carbide mixture. The mixture was rolled into a WC sheet using a doctor blade to adjust thickness.
Application
The 70-mm diameter pilot impellor test specimens for FGD were manufactured from Type 304 stainless steel (SS) (UNS S30400). A WC sheet was placed directly on the surface of the impellor. A flexible Ni-base alloy sheet was used as the top layer, as shown in Figure 2. Both sheets were placed on the impeller before vacuum brazing.
Vacuum Furnace
The brazing was performed in a vacuum furnace, shown in Figures 3(a) and (b), with a box chamber that included a rotary system. The rotary axis is located at the center of the box chamber, which is sealed with a non-contact, magnetic fluid seal that is clean and works in a high vacuum.
During the brazing process, the specimen with sheets was attached to the axis rotary system in the chamber. The chamber was then evacuated to 0.133 Pa and heated at a rate of 15 °C/min. During this process, the binders in the combined flexible sheets were evaporated at just under 400 °C. The Ni-base alloy sheet started to melt at 980 °C. Small pores formed by binder evaporation in the WC sheet provided capillary action that drew the liquid Ni into the WC sheet.10 The temperature was increased to 1,100 °C. The holding time at this temperature was determined from results of finite element method (FEM) simulation. Finally, the furnace was turned off and the specimen cooled to room temperature.
Lifetime Evaluation Test
A schematic of the impeller service life evaluation tester is illustrated in Figure 4. Impellers with and without the brazed WC-Ni layer were prepared. The initial weight of the specimens was measured with an electronic balance. Then the specimens were attached to the axis as shown in Figure 4. Aluminum oxide (Al2O3) is usually used as the erodent in wear tests due to its high hardness. An Al2O3 slurry was prepared with an average Al2O3 particle size of 150 μm. The slurry concentration was 10 wt%. The SS container was filled with 100 L of the slurry. The combined erosion and corrosion tests were conducted using 10 wt% sodium chloride (NaCl) solution and the Al2O3 slurry.
The impeller specimen was rotated by a 0.75-kW motor with the driving speed controlled at 300 rpm. For all impellers tested, the specimen was removed from the tester at 24-h intervals, cleaned using an ultrasonic bath, and air dried. The weight of each impeller was measured using an electronic balance to determine mass loss.
Results
The weight loss of each impeller after the test is shown in Figure 5. The weight loss of the impeller coated with the WC-Ni brazing layer was almost nil. The weight loss of the impeller that wasn’t brazed is significant as the specimen decreased 0.03 g after 96 h of testing time. By forming a passive film on the surface, Ni provided good corrosion protection properties. Sriraman, et al.14 reported that the formation of the oxide film promoted by WC content in the Ni improved corrosion resistance. Furthermore, the passive behavior of Ni became increasingly evident as the time of immersion increased. The {1 1 1}-oriented grains exhibit higher corrosion resistance than other orientations in Ni due to close-packed direction and the tenacity of the oxide layer in this orientation.15 For this reason, the Ni element contributed to the corrosion resistance of WC-Ni brazed coatings.
Conclusions
A WC-Ni brazing layer on the surface of the impeller for FGD was manufactured by conventional vacuum brazing with a rotary system in a furnace chamber with a magnetic seal. The rpm of the rotary brazing process was estimated from FEM simulation and that corresponded with the actual brazing process. The serviceable lifetime of the impeller with an applied WC-Ni brazing layer on the surface measured almost eight times longer than the unbrazed impeller.
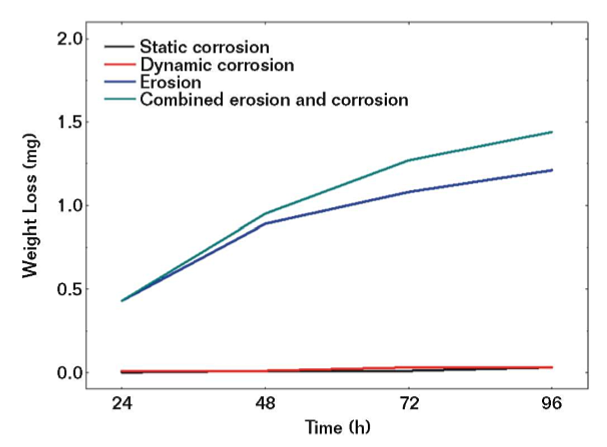
References
1 X.M. Li, D. Reinhoudt, M. Crego-Calama, “What Do We Need for a Superhydrophobic Surface? A Review on the Recent Progress in the Preparation of Superhydrophobic Surfaces,” Chemical Society Reviews 36 (2007): pp. 1,350-1,368.
2 H. Singh, et al., “Use of Plasma Spray Technology for Deposition of High Temperature Oxidation/Corrosion Resistant Coatings,” Mates. Corros. 58 (2007): pp. 92-102.
3 S. Lu, O. Kwon, “Microstructure and Bonding Strength of WC Reinforced Ni-Base Alloy Brazed Composite Coating,” Surface and Coatings Technology 153 (2002): pp. 40-48.
4 K. Van Acker, et al., “Influence of Tungsten Carbide Particle Size and Distribution on the Wear Resistance of Laser Clad WC/Ni Coatings,” Wear 258 (2005): pp. 194-202.
5 N. Espallargas, et al., “Cr3C2-NiCr and WC-Ni Thermal Spray Coatings as Alternatives to Hard Chromium for Erosion-Corrosion Resistance,” Surface and Coating Technology 202 (2008): pp. 1,405-1,417.
6 S.P. Lu, O.Y. Kwon, Y. Guo, “Wear Behavior of Brazed WC/NiCrBSi(Co) Composite Coatings,” Wear 254 (2003): pp. 421-428.
7 G.B. Stachowiak, G.W. Stachowiak, “Tribological Characteristics of WC-Based Claddings Using a Ball-Cratering Method,” Int. J. Refractory Metals and Hard Materials 28 (2010): pp. 95-105.
8 L.X. Gao, T. Liu, D.Q. Zhang, “Preparation of NiCrBSi-WC Brazed Coating and Its Microstructure Characteristics,” Surface Interface Analysis 45 (2013): pp. 767-772.
9 H. Klaasen, et al., “Reliability of Dual Compounds ‘Carbide Composite+Steel’ Produced by Diffusion Welding,” Int. J. Refractory Metals and Hard Materials 28 (2010): pp. 580-586.
10 E.J. Wentzel, C. Allen, “The Erosion-Corrosion Resistance of Tungsten-Carbide Hard Metals,” Int. J. Refractory Metals and Hard Materials 15 (1997): pp. 81-87.
11 E.J. Wentzel, C. Allen, “Erosion-Corrosion Resistance of Tungsten Carbide Hard Metals with Different Binder Compositions,” Wear 181-183 (1995): pp. 63-69.
12 M. Reyes, A. Neville, “Degradation Mechanisms of Co-Based Alloy and WC MetalMatrix Composites for Drilling Tools Offshore,” Wear 255 (2003): pp. 1,143-1,156.
13 A. Neville, et al., “Erosion-Corrosion Behaviour of WC-Based MMCs in Liquid-Solid Slurries,” Wear 259 (2005): pp. 181-195.
14 K.R. Sriraman, et al., “Corrosion Behaviour of Electrodeposited Nanocrystalline Ni–W and Ni–Fe–W Alloys,” Mater. Sci. Eng. A 460-461 (2007): pp. 39-45.
15 J.H. Chang, et al., “Corrosion Behaviour of Vacuum Induction-Melted Ni-Based Alloy,” Corros. Sci. 52 (2010): pp. 2,323-2,330.