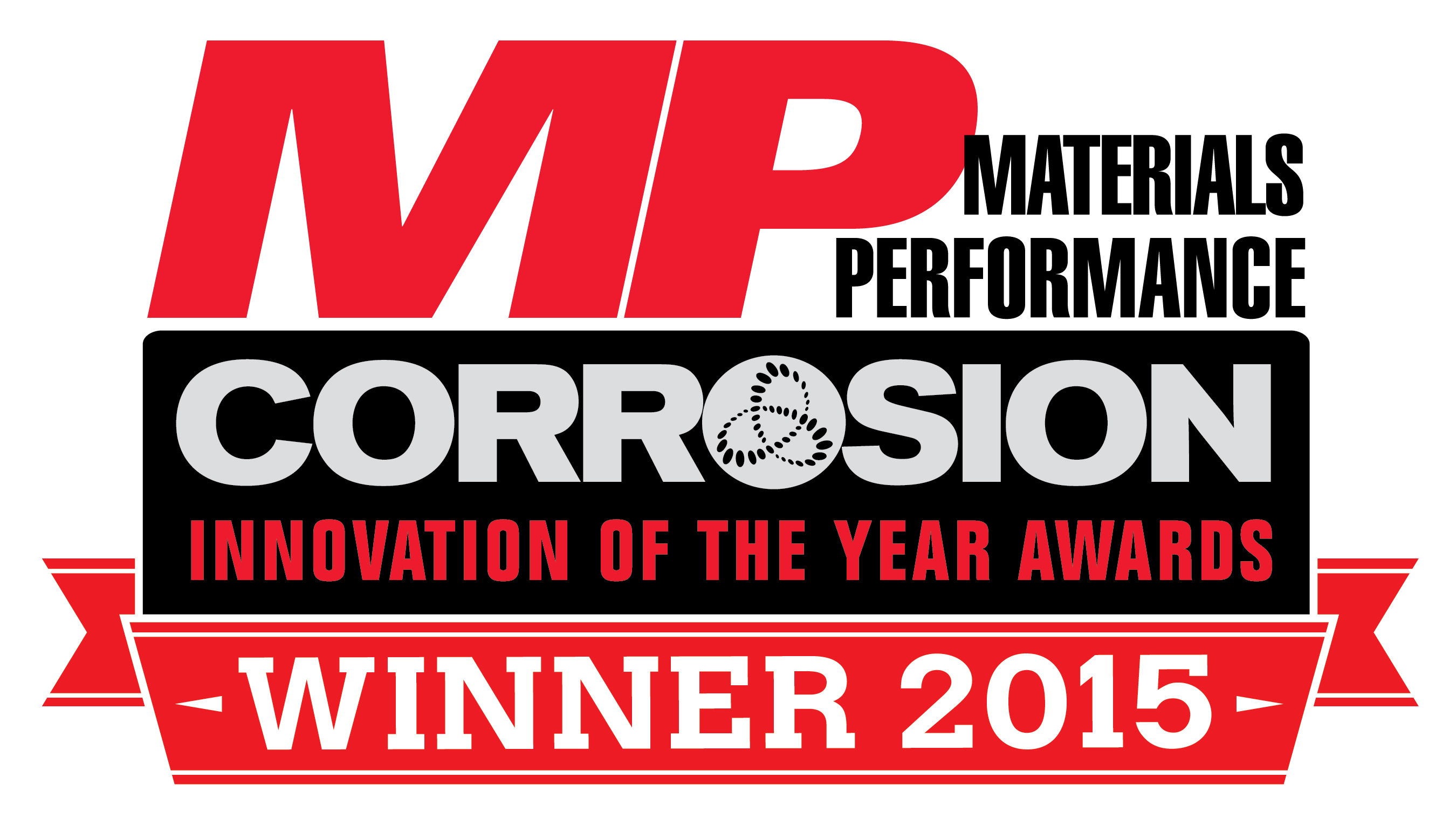
Researchers with Hempel A/S (Lyngby, Denmark) have developed a novel, organic zinc-rich primer coating technology that relies on a combination of zinc dust, hollow glass spheres, and a proprietary activator to provide excellent cathodic protection (CP) with greatly improved mechanical properties (crack resistance) and adhesion. This technology activates the zinc so that the zinc in the coating can be fully utilized to act as an anode. The use of glass spheres enables the coating to have a lower pigment volume concentration and more epoxy binder, which enhances coating adhesion. The unique corrosion byproduct of the activated zinc has a self-healing effect on the coating. The glass spheres block microcracks and also create an inhibitor effect by collecting insoluble complexes of zinc, oxygen, and chlorides on their surfaces and trapping these species in the coating so they don’t reach the surface of the steel.
Organic zinc-rich primers have been used since the 1960s and are an established method of corrosion protection for steel. These systems use high levels of zinc dust as a pigment in an organic binder (epoxy) to create a galvanic effect that protects the underlying steel substrate from corrosion. The zinc particles are more active than steel and act as anodes in the coating that corrode sacrificially instead of the steel when they are exposed to water, oxygen, and/or chlorides. Zinc-rich coatings are primarily used for corrosion protection of steel structures; and organic zinc-rich primers are often preferred over inorganic zinc-rich primers because they are less sensitive to surface preparation, over application, and humidity, which makes application easier. Experience in the field, however, has indicated that organic zinc-rich primers may not provide the level of CP that can be obtained from hot-dip zinc galvanizing or inorganic zinc silicate coatings, and can experience premature breakdown due to blistering and red rust formation. Additionally, organic zinc-rich primers may crack if over-applied, which leads to coating failures often seen on welds and corners.
Electrical conductivity and effective CP depend on the amount of the zinc dust used in a zinc-rich primer, says David Morton, director of Hempel’s Protective R & D Group. If the zinc content is too low, the organic binder will completely encapsulate the zinc dust. This results in limited or no contact between the zinc particles and, subsequently, electrical conductivity is limited, which reduces or eliminates CP of the steel. The amount of zinc dust also impacts the physical properties of the coating film such as adhesion, cohesive strength, and mechanical integrity—all important factors in determining the lifetime of the coating system. Attempts to improve the coating’s CP properties by using higher levels of zinc can have a negative impact on the mechanical and adhesion properties of the coating and result in premature coating failure. Although excessive zinc in the coating provides excellent CP because there is good contact between zinc particles and good conductivity, the adhesion to steel, cohesive strength, and mechanical properties usually will be deficient because the organic binder content is too low in relation to the zinc content. This is why the formulation of an organic zinc-rich primer is so important if the overall coating system is to be successful in providing corrosion protection through CP. From a coatings manufacturer’s point of view, Morton says, it’s very important to balance the coating formulation so it has reasonable adhesion and the best mechanical properties possible while retaining galvanic corrosion protection from the zinc.
Hempel’s research and development team studied ways to improve the effectiveness of conventional zinc-rich epoxy coatings and, in 2007, made an important discovery—while a typical standard organic zinc-rich epoxy coating contains ~80 wt% zinc, just one-third of the zinc actually contributes to corrosion protection. The research showed that only the zinc located in the 20 to 30 µm closest to the steel was consumed by the galvanic reaction in a zinc-rich coating with a dry film thickness (DFT) of 60 to 80 µm. About 60% of the zinc added to the primer was not used in a galvanic reaction.
To formulate a more efficient zinc-rich epoxy primer, the team spent more than 8,000 h in the lab, evaluated more than 800 prototypes, and applied coating to more than 3,000 test panels. Their efforts led to the development of an activated zinc-rich epoxy primer coating technology that incorporates tiny hollow glass spheres (~40 µm in size) and a special proprietary additive called an “activator.” With the addition of the glass spheres and an activator that improves the conductivity of the entire coating matrix, the volume of zinc dust required (relative to the epoxy resin) for effective CP is reduced while maintaining a dry film weight percent of zinc dust that complies with current standards. With the lower zinc volume in the coating, more of the epoxy binder is available, Morton explains, which promotes better adhesion properties and mechanical performance. Because of the synergy of these components, the coating delivers three methods of corrosion protection: a galvanic effect, a barrier effect, and an inhibitor effect.
For galvanic protection, the activator increases the zinc’s ability to carry the corrosion current throughout the coating although the zinc particles are not in direct contact with each other, which greatly improves CP of the steel. The coating’s barrier properties and self-healing characteristics are delivered by the corrosion product of the zinc. Typically, Morton says, the corrosion product of a zinc-rich primer is zinc oxide (ZnO). In the activated zinc-rich primer, he notes, the corrosion product created is an insoluble salt—zinc chloride hydroxide hydrate. This insoluble salt forms a uniform protective layer on the surface of the primer, which acts as a barrier that blocks water, oxygen, and chlorides from reaching the steel surface. Additionally, byproduct from the rapidly corroding activated zinc fills any microcracks in the coating, essentially enabling the coating to heal itself.
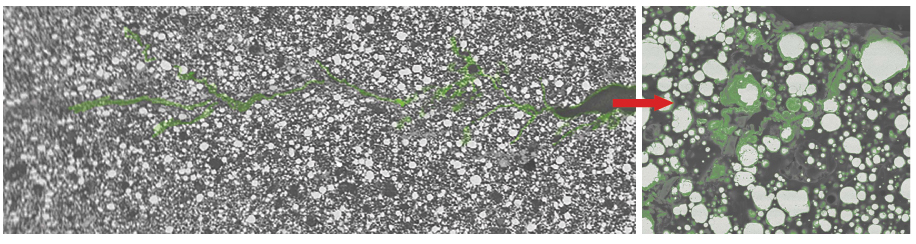
The addition of the hollow glass spheres enhances the coating’s physical properties. The spheres improve the film’s crack resistance by blocking the propagation of microcracks, and they contribute to the coating’s low permeability as well.
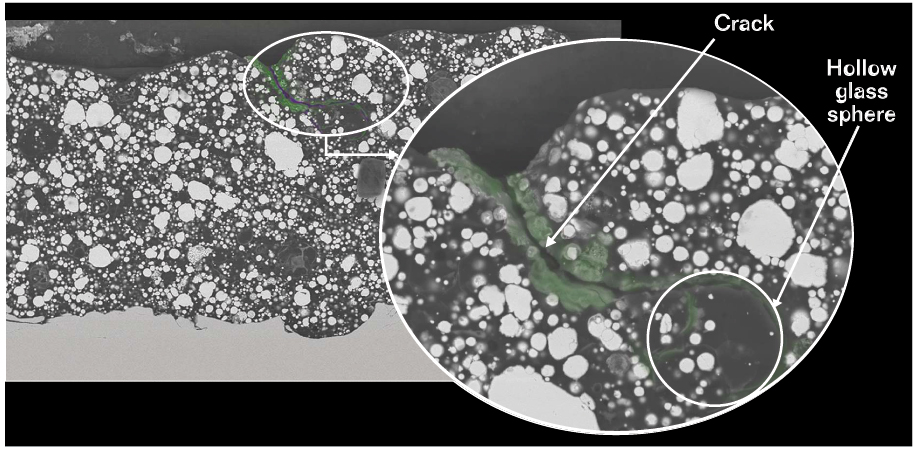
Additionally, the glass spheres are important contributors to the coating’s inhibitor effect. “We can observe an accumulation of insoluble complexes of zinc, oxygen, and chlorides on the surfaces of the glass spheres that become part of the coating instead of reaching the steel substrate,” Morton explains. The zinc corrosion product created during galvanic corrosion also acts as environmental scavenger by capturing chloride ions as they diffuse into the coating from the environment.
Morton comments that the activated zinc-rich epoxy primers are normally applied as part of a three-coat protective coating system that also includes an epoxy intermediate coat and a polyurethane (PUR) topcoat, which is standard for coating protection of outdoor steel structures such as bridges, offshore oil platforms, coastal power plants, and refineries in industrial and marine environments with high relative humidity and aggressive atmospheres, including coastal and offshore areas with high salinity (described in ISO 129441 as a C5 environment). Samples coated with the activated zinc-rich epoxy primer with a DFT of ~100 µm were exposed in an accelerated salt spray exposure test (per ISO 92272) for 1,259 h and passed without signs of rust. Samples coated with a three-coat system with the activated zinc-rich primer, epoxy intermediate, and PUR topcoat passed a neutral salt spray test (per ISO 12944 for the C5 category) after being exposed for 1,440 h
The activated zinc-rich primer also showed good results when tested for adhesion (ISO 46243) and crack resistance (NACE TM03044). Scanning electron microscope (SEM) images were taken to evaluate the degree of cracking and self-healing in the activated zinc-rich epoxy primer. It was possible to observe the hollow glass spheres stopping the development of cracks, as well as the zinc corrosion product filling the empty space of the microcracks. To qualitatively evaluate the chemical composition of the deposit formed around the glass spheres in the primer, energy dispersive x-ray (EDS) elemental mapping studies were done. Chloride atoms were detected in the zinc salts that surrounded the hollow glass spheres, suggesting that the spheres work as a surface for the deposition of salts, which provides an inhibitor effect.

The new activated zinc-rich primer technology was launched in 2014 as part of the AvantGuard† product series.
Contact David Morton, Hempel—e-mail: dmor@hempel.com.
References
1 ISO 12944-5:2007, “Paints and varnishes—Corrosion protection of steel structures by protective paint systems—Part 5: Protective paint systems” (Geneva, Switzerland: ISO, 2007).
2 ISO 9227:2012, “Corrosion tests in artificial atmospheres—Salt spray tests” (Geneva, Switzerland: ISO, 2012).
3 ISO 4624:2002, “Paints and varnishes—Pull-off test for adhesion” (Geneva, Switzerland: ISO, 2002).
4 NACE Standard TM0304-2004, “Offshore Platform Atmospheric and Splash Zone Maintenance Coating System Evaluation” (Houston, TX: NACE International, 2004).
†Trade name.