Offshore wind turbines are subjected to harsh marine environments, which can cause detrimental problems to their integrity due to corrosion. Offshore wind energy will play an important role in achieving the net zero emissions target by 2050, hence the long-term protection of these structures is of high importance. In this work, the formation of microcapsules loaded with corrosion inhibitor liquid is presented. Different variables such as agitation rates, times, and surfactants were evaluated to achieve optimum size. The incorporation of the microcapsules into waterborne paint could offer a corrosion solution to these structures when comwith thermally sprayed coatings.
Organic metallic structures have an average lifetime of 20 to 25 years. They consist of four different zones: the buried, the submerged, the tidal/splash, and
the atmospheric. According to NACE International, in 2013 the global corrosion cost is estimated to be U.S. $2.5 trillion,1 constituting a major economic problem. Hence, protection from corrosion is essential.
Each zone is protected either with cathodic protection (CP), or with a combination of CP and coatings. More specifically, the protection of the atmospheric zone, which is the aim of this research, does not allow the application of submerged anode CP due to the lack of continuous electrolyte (seawater).
Due to this limitation, the application of protective coatings was chosen. As an additional protective coating, a thermally sprayed zinc (TSZ) of 85 wt.% Zn and 15 wt.% Al wire alloy composition coating was selected to be applied on the metallic substrate. The latter is a well-known and widely used technique in corrosive environments. It is noticeable that the combination of both Zn and Al provide greater corrosion resistance properties.2
As a suggested solution to corrosion on the atmospheric zone, this research focuses on the development of a multilayer coating system. Thermally sprayed Zn/Al sacrificial coating in conjunction with an epoxy-based marine coating enriched with self-healing microcapsules will lead to a novel autonomous extrinsic self-healing anticorrosion system.
Even if the idea of self-healing coatings is not new and there are a few studies on this topic, the industrial application of them in coatings is very limited or non-existing.3 Silanes are used as corrosion inhibitors due to their chemistry, which protects the underlying substrate.4
After the incorporation of the microcapsules in the selected paint, artificial scratches will be incorporated onto the coupons. As a result, once the microcapsules rupture and the core material is released, its contact with the environmental conditions will initiate the self-healing reaction.
The development of novel coating systems with application on wind turbines will have a significant impact on the reduction of their maintenance and replacement costs, ensuring protection against corrosion, extension of their service life, and reduction of catastrophic incidents that could have devastating social, environmental, and financial consequences.
Experimental Procedure
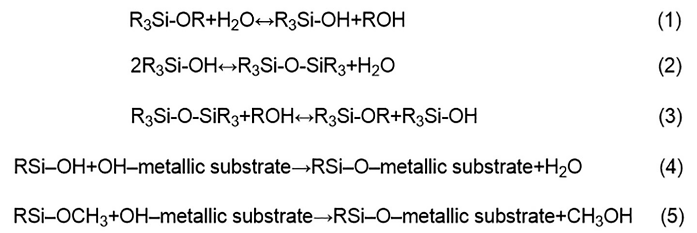
All the materials and reagents were used as received. Carbon steel (CS) (S355) commonly used in the offshore industry was used as a substrate and was coated with TSZ.
The regents consisted of thermally sprayed 85 wt.% Zn, 15 wt.% Al wire alloyed composition. 3-octanoylthio1-propyltriethoxysilane, ethanol (CH3CH2OH), nitric acid (HNO3) (65%), polyvinyl alcohol (C2H4O)n, dichloromethane (CH2Cl2), polystyrene (C8H8)n1, sodium dodecylbenzene sulfonate (SDBS) (C₁₈H₂₉NaO₃S), Silwet,† and acacia gum.
Silicone polymers (polysiloxanes) are a product of polymerization. Hydrolysis is the initial step of this process, followed by condensation, and lastly phase separation, from which sol or gel may appear. More specifically in the organoalkoxysilane, the alkoxy groups are replaced by hydroxyl groups in acidic conditions.5 The formation of silane bridges (Si-O-Si) are the result of the condensation.6 Figure 1 represents these consequent reactions, as well as reactions with the substrate.
Acidic conditions were selected for faster and increased formation of hydroxyl groups and at the same time for slowing down the self-condensation reaction.7 The nature of silane, pH, temperature, water/silane ratio, and silane/solvent ratio affect the kinetics of the
described reactions.
In this research, pH was adjusted to around 4 with HNO3 solution. However, when the hydrolysis medium was 80/20 EtOH/H2O, the result was a transparent liquid with some tiny oily droplets, while in a 50/50 medium there were many oily droplets.
The synthesis of corrosion inhibitor and of the polystyrene microcapsules are described in an AMPP paper,8 using the solvent evaporation from a double emulsion method. For the formation of the emulsions, magnetic stirring was used. Three different emulsifiers were evaluated: siloxane copolymer, acacia gum, and SDBS. From these, SDBS was selected as the obtained microcapsules had the optimum size diameter. Moreover, tests with different agitation rates (400, 500, 600, and 700 rpm) were conducted.
Polystyrene was selected as the shell, because it is an inexpensive, widely used, chemically inert, and quite rigid material. These properties make it an ideal shell for the self-healing core material. It is resistant to water and to many bases and acids, but it can be easily dissolved from many organic solvents, such as acetone. Moreover, its compatibility with marine paints should be considered for the selection of the marine paint into which the microcapsules will be later mixed. Hence, a marine paint low in volatile organic compounds will be used.
For the characterization of the microcapsules, scanning electron microscope (SEM) and optical microscope (OM) were used. Microcapsules’ surface, microstructure details, and diameter sizes were obtained. As a variety of sizes was detected, the dynamic light scattering (DLS) technique was also used to determine the size distribution profile of the microcapsules.
Results And Discussion
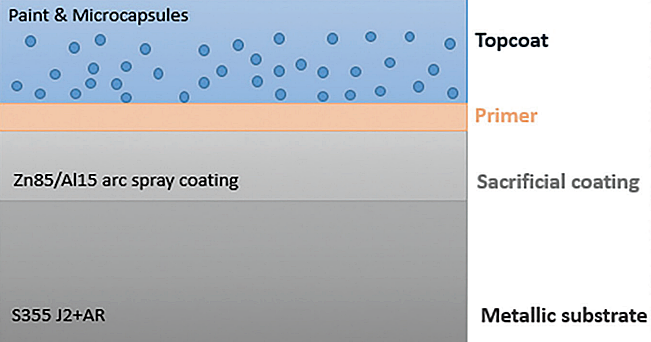
Figure 2 represents the new coating system that will be developed. The metallic substrate is CS (S355), followed by TSZ (85 wt.% Zn, 15 wt.% Al wire alloyed composition) coating. After the application of a primer for better adhesion of the paint, the topcoat will be applied. This will consist of marine epoxy paint enriched with the formatted microcapsules.
After the separation of the microcapsules from the liquid medium and their cleaning with ethanol, the product was allowed to fully dry. A white and fine powder of around 5 g was obtained, as observed in Figure 3(a), top. After examination under OM [Figure 3(b), top] and SEM, mononuclear microcapsules with a single inner cavity were observed. The DLS technique was used, and a variety of diameter sizes was observed.
From this analysis, it was decided that the agitation rate to obtain 80 to 100 μm capsules is between 400 and 500 rpm, as observed in Table 1. The successful encapsulation of the silanol was confirmed from the rupture of the polystyrene shell and the release of the liquid in Figure 3(c), top.
It is notable that when higher speeds were used, 700 and 800 rpm, nanoscale microcapsules were formed. This observation can be related to surface tension and shear force phenomena or silanol
concentration.9 Finally, the selection of the surfactant for the emulsion formation as well as the addition of the reagents into the reaction solution had a crucial importance for the shape and size of the microcapsules.
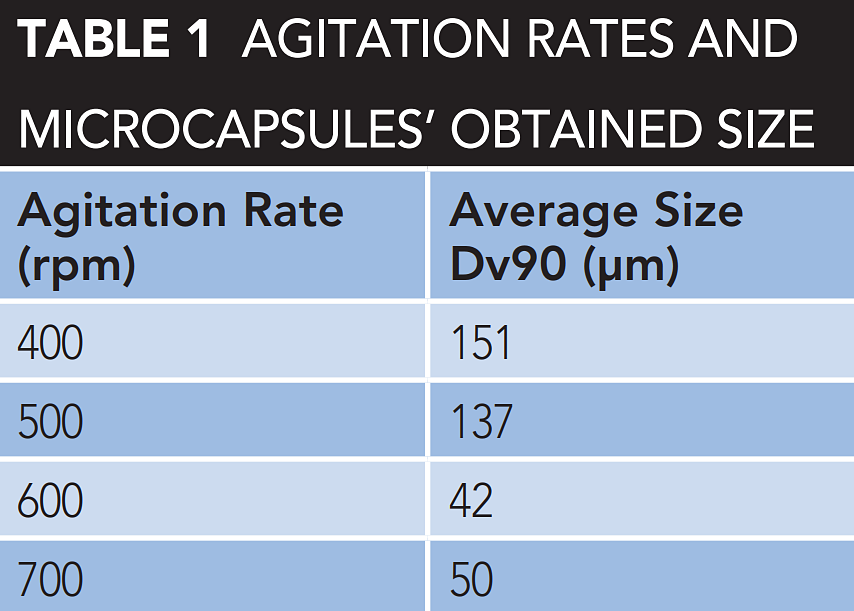
Conclusions
The microcapsules that were formed from the solvent evaporation from a double emulsion technique had a variety of sizes. The optimum size was achieved when 400 and 500 rpm magnetic stirring was implemented. Spherical, one-core and loaded with corrosion inhibitor microcapsules were developed. Images from OM and SEM showed a spherical and well-built outer shell of the microspheres.
To confirm that they were loaded with corrosion inhibitor liquid, some of them were intentionally ruptured with a spatula. The release of liquid confirmed the successful experimental procedure.
Sieves with specific diameter will be used for the separation of the various sizes of microcapsules prior to their incorporation into the marine paint. Exposure in corrosive conditions and monitoring of the chemical reactions will give valuable information regarding the anticorrosive and self-healing properties of the newly developed system in this ongoing research.
Acknowledgements
This publication was made possible by the sponsorship and support of Lloyd’s Register Foundation, a charitable foundation helping to protect life and property by supporting engineering-related education, public engagement, and the application of research: http://www.lrfoundation.org.uk.
The work was enabled through, and undertaken at, the National Structural Integrity Research Centre, a postgraduate engineering facility for industry-led research into structural integrity established and managed by TWI through a network of both national and international universities. Authors gratefully acknowledge financial support from the Centre for Doctoral Training in Innovative Metal Processing funded by the U.K. Engineering and Physical Sciences Research Council.
† Trade name
This article was originally published in the January 2023 print edition of Materials Performance. Republished with permission.