Closed hydronic loops are of critical importance in many applications including industrial process equipment as well as heating, ventilation, and air conditioning systems. Molybdate and nitrite have been used for decades to control corrosion in closed loops, along with borate as buffer. However, each chemical has its limitations. Because of these drawbacks and increasing environmental regulations, new nontoxic corrosion inhibitors are needed.
Here we present a next generation nontoxic closed loop corrosion inhibitor that contains no nitrite, heavy metal, P, B, or filming amine. Laboratory feasibility experiments show excellent corrosion inhibition results <0.05 mm/y (<0.2 mpy) on mild steel.
Closed cooling loops are encountered across a variety of industries where cooling is critical to operations and operators cannot tolerate the variability typically seen in open cooling towers. Most industrial plants will contain some number of closed cooling loops; closed loops can be found in refineries, petrochemicals, steel making, and microelectronics. Because closed cooling loops can run with little maintenance for long periods of time, they can become a neglected part of a customer’s water treatment system.
This neglect can lead to significant issues that can negatively impact heat transfer efficiency in the cooling process. The most common issues in closed cooling loops are corrosion and microbial growth.1-2 In terms of water quality, the main driver for corrosion in closed loops is chloride concentration. Most closed loops have a chloride concentration <50 ppm.
Typical temperatures encountered in closed loops vary, but in general temperatures are 35 to 80 °C. Closed loops are constructed mostly with carbon steel (CS), stainless steel, and copper alloys; however, the use of aluminum alloys in closed loops is becoming more prevalent. Closed loop volumes can vary significantly; in heating, ventilation, and air conditioning applications volumes can be as low as 10 gal (37.85 L), whereas some refineries have closed loop volumes of several million gal.
Corrosion inhibition in closed loops is predominantly treated using nitrite and molybdate-based corrosion inhibitors,3 although organic-based closed loop corrosion inhibitors have been reported.4-5 Nitrite provides excellent corrosion inhibition performance over a wide range of temperatures and chloride content. Nitrite acts as an anodic corrosion inhibitor and functions without the need of dissolved oxygen.
Despite excellent corrosion inhibition performance, nitrite does have several limitations. The biggest potential issue with nitrite in closed loops is its biodegradability in the presence of microbiological organisms. This biodegradability is an issue for two reasons; first, the nitrite is consumed by microbiological growth, which increases the risk of loop corrosion, specifically pitting corrosion, if nitrite levels are decreased significantly. Second, microbial growth can easily get out of control since the nitrite is an excellent food source that can encourage even more microbial growth, which can lead to microbiological induced corrosion. The potential for microbial growth in closed loops treated with nitrite can also lead to significant water costs.
For example, closed loop operators’ standard operating procedure is to keep microbial content below a certain threshold. When/if the nonoxidizing biocides are ineffective at reducing microbial counts, the loop must be drained and refilled with clean makeup water. Depending on the extent of microbial problem in the loop, this makeup water cost can be quite significant. Nitrite is also toxic, so any nitrite-based corrosion inhibitor suffers from a safety, health, and environment (SH&E) perspective. Molybdate is also used as a corrosion inhibitor in closed loops.
Molybdate does not have the large application window that nitrite has; molybdate struggles to inhibit corrosion when chloride levels are >15 ppm. Molybdate is also much more expensive than nitrite. Additionally, in some regions there are very strict discharge limits on Mo, which limits where MoO4 can be used as a corrosion inhibitor. An advantage of molybdate closed loop corrosion inhibitors is that microbiological growth does not consume molybdate.
Closed loop corrosion inhibitor formulations must also include a buffer to keep loop pH high in case of process leakage into the loop. Historically, borates have been formulated into closed loop corrosion inhibitors due to their low cost and appropriate buffering range (pKa). Currently, however, borates are classified as a substance of very high concern by the European Union. Borates have been classified as a toxin for human reproduction. As such, water treatment companies are removing borate from their product lines due to these SH&E concerns around borate.
We present here a novel anchoring corrosion inhibitor derived from carboxylate (AC/DC) for CS that does not contain nitrite, heavy metal, boron, phosphorus, or filming amine. An inert fluorescent tracer was blended into the formula for advanced product monitoring and control. The AC/DC-based corrosion inhibitor package meets the characteristics described above as well as additional features outlined below.
Experimental Procedure
Synthetic Cooling Water Chemistry 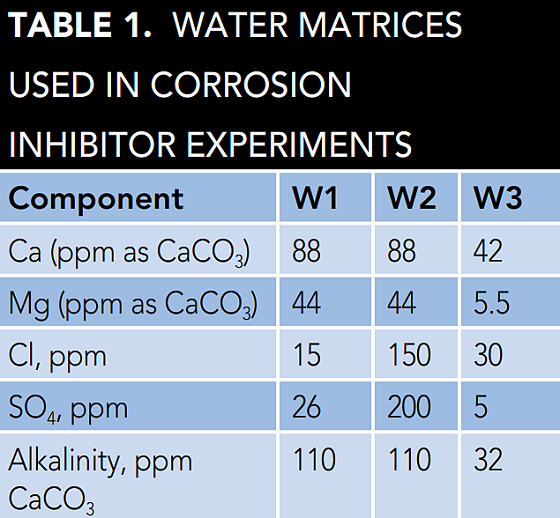
Two water matrices were used for the tests described in this article. Naperville, Illinois, USA tap water (Table 1, W1) is a low corrosion stress water due to relatively high calcium and alkalinity, and low concentration of corrosive ions such as chloride and sulfate.
To increase the corrosivity of the water, and stress the corrosion inhibitors, additional chloride (sodium chloride [NaCl]) and sulfate (sodium sulfate [Na2SO4]) were added into Naperville tap water to reach 150 ppm chloride and 200 ppm sulfate (Table 1, W2). A chloride concentration of 150 ppm and a sulfate concentration of 200 ppm would represent 80% of the makeup water in the United States. A third water matrix was used to simulate the makeup water typically used for closed loop cooling water applications (Table 1, W3).
Jar Tests
Corrosion inhibition performance was evaluated using stirred jar tests at 40 °C and 60 °C. Glass jars were filled with water and were then dosed with the corrosion inhibitor to be evaluated. Total mass in each jar was 400 g. Fresh mild steel coupons were submerged under the liquid level and were suspended using polytetrafluoroethylene tape.
The jars were sealed and placed in a heater shaker water bath that was temperature-controlled. The jars were heated and shaken at 60 rpm for 17 d after which the coupons were removed and analyzed. General corrosion rates were calculated gravimetrically; coupons were also inspected for signs of localized corrosion.
Pilot Closed Loop Testing
The pilot closed loop testing apparatus is shown in Figure 1. A coupon rack was built on a recirculating water bath. Typically, the testing temperatures were 10 °C, 60 °C, and 80 °C to mimic both cooling and heating loops. After the desired runtime, coupons were removed, and general corrosion rates were calculated gravimetrically; coupons were also inspected for signs of localized corrosion. Instantaneous corrosion rates in the pilot closed loops were also monitored using inline CS linear polarization resistance (LPR) probes.
Pilot closed loop tests were also performed using filming amine, oleylpropylenediamine (OPDA). The OPDA-based formulation also contained tertiary amine, azole, and an organic surfactant to increase OPDA solubility.
The pH of the OPDA pilot closed loop experiment was 8.8. The OPDA concentration investigated (64 ppm actives) was greater than the typical dose of OPDA in closed cooling applications. A high dose of OPDA was chosen to thoroughly evaluate the comparative performance of OPDA vs AC/DC. The AC/DC dose was 2,500 ppm as product, which is consistent with typical dosage of conventional closed cooling corrosion inhibitors.
Results
Jar Tests
The AC/DC corrosion inhibitor was evaluated first in jar tests. Jar tests provide a quick test, albeit with slightly higher corrosion rates than are typically observed in the field. Jar test results are shown in Table 2.
Tests were conducted at 40 °C and 60 °C with W2 and W3. In tests performed using W3, AC/DC displays excellent corrosion inhibition at 40 °C and has good performance at 60 °C. Even in the highly corrosive W2 synthetic closed loop water (Cl– = 150 ppm), AC/DC displays excellent performance. There were no signs of localized corrosion in any of these tests. 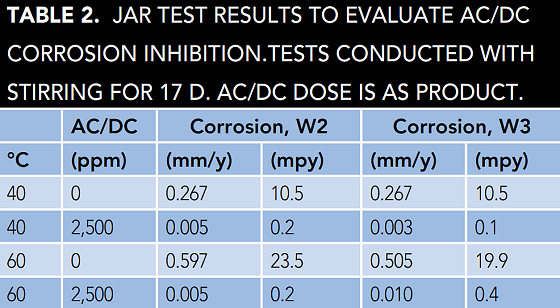
Pilot Closed Loop Test Results
Pilot closed loop testing was used to reproduce the positive jar test results in a system that better simulates field conditions. A photograph of the pilot closed loop is shown in Figure 1 (top). Pilot closed loop experiments are designed to simulate field conditions, but also stress potential new chemistries.
For example, the pilot closed loop testing reported here is fully oxygenated, at higher chloride concentrations than would exist in field loops (W2), and at a lower pH (8.5 at the end of the experiment) than typical closed loops. Therefore, any chemistry that succeeds in these difficult test conditions should perform well in field closed loops where dissolved oxygen is minimal, chloride concentration is <50 ppm, and pH values are >8.5.
Pilot closed loop test results are shown in Table 3. Experiment runtimes are ~30 d unless severe corrosion has taken place. Table 3 shows excellent AC/DC corrosion inhibition performance in W2. Photographs of the mild steel coupons from all entries in Table 3 are shown in Figure 2. 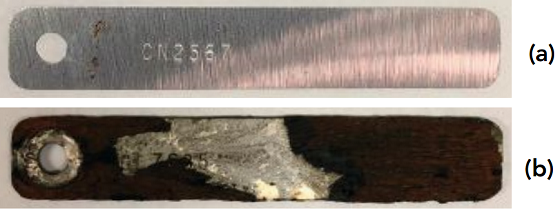
Filming amines are used industrially to inhibit corrosion in closed loops. Filming amines, in particular OPDA, have been used quite successfully to inhibit corrosion in boiler condensate systems. We thought it important to compare the corrosion inhibition performance of AC/DC with an OPDA-based formulation. Further details on the OPDA-based formulation and pilot closed loop experiment are described in the Experimental Section. Results of this experiment are shown in Table 3, and a photograph of the mild steel coupon is shown in Figure 2(b).
Corrosion inhibition results were quite poor for the filming amine-based formulation. Filming amines are excellent corrosion inhibitors in low chloride waters (such as boiler condensate); however, performance is poor when chloride concentration is increased. The failure of OPDA in these closed loop experiments demonstrates the value in designing experiments using highly corrosive waters. The authors’ aim is to design a robust chemistry with a broad application window capable of handling aggressive conditions including high chloride and high heat flux.
Our investigations into new closed loop corrosion inhibitors revealed that the main driver for corrosion in closed loops is chloride. Ideally, operators would fill their loops with demineralized water; however, the reality is that more closed loop operators are filling their loops with higher chloride waters as water scarcity and water recycle become more prevalent. As such, a corrosion inhibitor that can tolerate the higher chloride content of today’s loops is desirable. 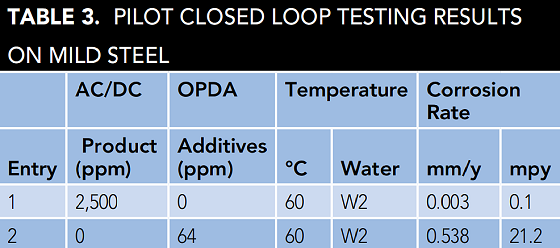
By using LPR probes installed in the recirculating loop of the pilot closed loop, it was possible to evaluate the impact of different chloride/sulfate concentrations on incumbent corrosion inhibitors (nitrite and molybdate) as well as AC/DC. The corrosion data from these chloride tolerance experiments are shown in Figure 3. The first water investigated was W2 (150 ppm chloride, 200 ppm sulfate); in Figure 3, the data to the left of the dashed line was collected using W2.
As expected, based on the jar testing and pilot closed loop testing reported earlier, AC/DC showed excellent corrosion inhibition results using W2. The nitrite-based corrosion inhibitor also displayed excellent corrosion inhibition using W2. The molybdate-based incumbent corrosion inhibitor, however, displayed very poor corrosion inhibition, exhibiting unacceptable corrosion rates >0.025 mm/y (1 mpy). The chloride and sulfate content of the loop was then increased to 500 ppm chloride and 667 ppm sulfate (right side of dashed line).
The molybdate-based treatment continued to display very poor performance with corrosion rates exceeding 1.27 mm/y (50 mpy), whereas the nitrite-based treatment continued to exhibit excellent corrosion inhibition in the higher chloride water. The AC/DC program did display a slight increase in corrosion rate; however, corrosion rates remained <0.013 mm/y (0.5 mpy) even in highly corrosive water with a Cl–/SO42- concentration of 500 ppm/667 ppm, respectively. It should be noted that digital recording of the corrosion rate of AC/DC was lost after 1OCT; however, manual data recording showed the final corrosion rate by LPR on 3OCT was 0.5 mpy. 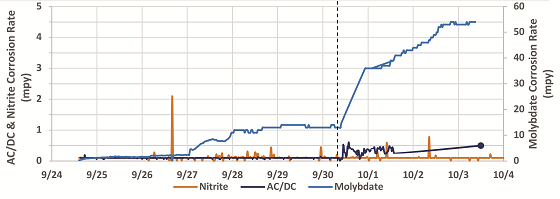
Conclusions
Closed cooling loops are a critical component of most industrial plants. The incumbent closed loop corrosion inhibitors are based on toxic components such as nitrite, molybdate, and borate that are coming under more SH&E scrutiny. New closed loop corrosion inhibitors are necessary that maintain corrosion inhibition performance while not containing toxic components. A novel AC/DC inhibitor was developed to mitigate corrosion in mild steel closed loops. AC/DC was formulated with azoles for copper alloy corrosion control. AC/DC does not contain nitrite, heavy metal, boron, phosphorus, or filming amine.
Data presented in this report show that AC/DC offers superior corrosion inhibition performance when compared to incumbent closed loop corrosion inhibitors based on molybdate and filming amines.
References and About the Authors