North American shale operations consist of several hundred well pads tied to central processing facilities by a network of gas and liquid pipelines. At the well pad, multiphase fluids from each well pass through a choke before entering a high-pressure (HP) separator. Liquids either pass directly to a liquids flowline or to a low-pressure (LP) separator, heater treater, and into storage tanks. Overhead gas from the HP separator is metered before entering the gas system, through 8-in (203-mm) flowlines. The normal operating pressure is 5.5 MPa. The carbon dioxide (CO2) concentration is 1 to 5 mol%, but typically 2 mol%. The hydrogen sulfide (H2S) concentration in the gas can reach 80 ppm but is usually <20 ppm. The gas is not typically inhibited; but if the well contains H2S, it is chemically treated by injecting a monoethanolamine (MEA) triazine H2S scavenger into a purpose-built flow loop to reduce the H2S concentration so it meets the pipeline specification of <4 ppm. High-H2S wells are treated using an Ultrafab† H2S mitigation unit. Gas velocities vary but often drop to 0.1 m/s (0.3 ft/s) as the well declines. Flow from several wells can be directed into a single flowline and compression can be used during the life of a well to boost production. The pipeline specification limits the gas temperature to 120 °F (49 °C) but most wells operate between 80 to 100 °F (27 to 38 °C).
Materials of Construction
The pipelines were fabricated from API 5L1 Grade X52 PSL2 electric-resistance welded (ERW) carbon steel (CS). The girth welds were made in accordance with API 11042 using shielded metal arc welding (SMAW), E6010 welding rod for the root pass, and an E8010 electrode for the hot pass and fill. The materials were not ordered to sour service requirements for API 5L Annex H. The girth welding procedures were not qualified to NACE MR0175/ ISO 151563 hardness requirements. The longitudinal welds had a postweld heat-treatment (PWHT) but girth welds were as-welded.
Gas Leak
A leak occurred in an 8-in gas flowline at a low point at the bottom of a decline where the pipe passed through a bore made for a dry river crossing. A circumferential crack passed through the pipe wall, causing the pipe to part when it was removed from the bore (Figure 1). There were multiple crack initiation sites on the inside of the pipe close to the girth weld. There was no significant wall loss due to corrosion, although shallow pitting was observed. There was an accumulation of solids close to the fracture, and some liquids were recovered.
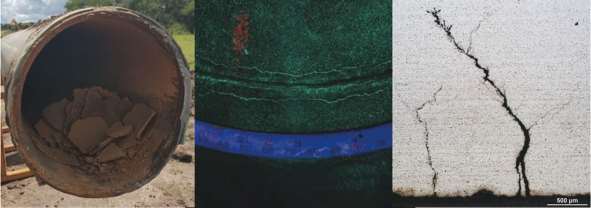
Girth welds upstream and downstream of the leak site were examined by wet fluorescent magnetic particle testing (WFMT). Twenty girth welds were found to be cracked. While the fractured weld was at the low point, the other cracked welds were all on the downstream side of the low point as the pipe rose up the incline. No welds were cracked on the downhill portion of the line that was upstream of the leak. At the low point, cracking was almost completely around the full circumference of the pipe; however, the cracking was reduced in extent and restricted to the lower quadrant on the incline. The pattern of cracking was consistent with liquid slugging up the incline.
Sectioning revealed multiple branched intergranular cracks typical of environmentally assisted cracking. All cracks were in the weld area. The majority of cracks were in equiaxed parent metal close to the heat-affected zone (HAZ), but weld metal and the HAZ were also cracked. The longitudinal ERW seam, which had a PWHT, was not cracked and neither was the parent metal away from the weld.
The maximum hardness recorded in the cracked welds was 201Hv10. All values were well below the NACE MR0175/ISO 15156 hardness limit. The H2S partial pressure of untreated gas at the maximum allowable operating pressure (MAOP) was 0.19 kPa. The flowline typically operated at half of the MAOP. If H2S scavenging was interrupted for some reason, the service condition would not be sour.
Magnetite (Fe3O4), goethite [FeO(OH)], siderite (FeCO3), and wustite (FeO) were found close to the cracks, but no mackinawite or iron sulfide by arsenide test was seen. Solids found at the fracture site included organosulfur compounds and ammonium salts. Sulfur and phosphorous were detected in the cracks. Percent levels of MEA and carbonate were found in pig inspection returns. Sulfur and phosphorus implicate the chemical treatment package and its reaction products. Ammonium carbamate (CH6N2O2) is a reaction product of MEA and CO2.
Eight other pipelines operating with low flow were destructively examined by sectioning at a low point. All showed intergranular stress corrosion cracking (IGSCC) at girth welds. Cracks found in seven of the pipelines were shallow (<200 µm), but IGSCC had penetrated 40% through the pipe wall in one pipeline. IGSCC was also found in flow loops that were destructively examined. The deepest cracks found in flow loops initiated from linear welding defects at the circumferential welds that joined elbows to straight sections. Approximately nine months after the first leak, a second pipeline leaked at a girth weld. The recovered spool displayed similar features to those described previously, which are typical of IGSCC (Figure 2).
Stress Corrosion Cracking
SCC requires a tensile stress and a corrosive environment. The tensile stress is usually residual stress from welding but also can be caused by mechanical damage or cold forming. SCC can be transgranular or intergranular and is found over a limited potential range in environments that promote passive behavior of the material. Cracking occurs in the active-to-passive transition and in the transpassive zones (Figure 3). Only a few environments are known to cause IGSCC in CS; amines (MEA, diethanolamine [DEA], and methyldiethanolamine [MDEA]), carbonate/bicarbonate, caustic, nitrates, phosphates, gas liquor, and anhydrous methanol.
Hydrogen Sulfide Scavenging Reaction
H2S is removed from the gas by scavenging with MEA triazine. The chemical treatment package consists of MEA triazine plus 6% MEA and an organophosphate scale inhibitor. The triazine reacts with two molecules of H2S to form dithiazine and 2 MEA. Under certain conditions, dithiazine polymerizes to amorphous dithiazine and ejects additional MEA. The pH of spent triazine is 12.5.
The scavenger is directly injected into flow loops to improve contact time and spent chemical scavenger freely enters the flowline. The injection rate is 2 to 3 gal/d (7.6 to 11.3 L/d) for a standard well. Short flowlines are not pigable.
Amine and Carbonate/Bicarbonate Stress Corrosion Cracking
Amine SCC was first recognized at refineries in 1951 in amine units used for CO2 and H2S removal. Cracking was slow, and could take from 5 to 15 years. It was more severe when using MEA vs. DEA or MDEA as a scavenger. Following a refinery disaster in the 1980s that resulted in several fatalities, amine SCC was studied extensively. Ironically, that failure was caused by sulfide stress cracking (SSC) of a weld repair due to a leak caused by amine SCC.
Amine cracking is always intergranular and is most prevalent at ~130 °F (54 °C). Gutzeit and Johnson4 reported cracks between 100 and 140 °F (37.8 and 60 °C) in MEA units. Hughes5 reported cracks in MEA storage tanks at ambient temperatures. API 9456 advises mitigation in solutions containing >2% MEA and operating at ambient temperatures and upward.
Cracking does not occur in pure MEA solutions; it requires the presence of CO2 and/or heat stable acid salts (formates, acetates). Cracking is more prevalent in lean amine but also occurs in rich amine. Cracking is slightly affected by the concentration of MEA, although most refineries operate with ~25%. SCC occurs at potentials between –0.5 and –0.8 V at pH 8 to 11. Amine cracking is unaffected by the hardness of the steel but low-carbon, higher-yield-strength steels appear to be more susceptible.
MEA SCC has been successfully prevented by PWHT. Detection methods include WFMT and shear wave ultrasonic testing, but cracks are very fine and difficult to find. Radiography can detect severe cracks but early cracking may be missed.
Parkins and Foster7 proposed that MEA SCC is a form of carbonate/bicarbonate cracking, and that amine is just a means of producing pH values in a range where CO2 will form a solution of bicarbonate/carbonate. This dependence is shown in Figure 4.
MEA reacts with CO2 to form a mixture of carbamate, bicarbonate, and carbonate. Cracking is worsened by the presence of heat-stable acid salts, acetate, formate, and glycolate following the oxidation of MEA if the process gas contains traces of oxygen. In shale gas, oxygen can enter through the vapor recovery system.
Carbonate cracking is found in refinery fluid catalytic cracking units (FCCUs) when the pH of the liquor goes above 8.0 and the carbonate concentration exceeds 100 ppm. Cracking is reported in equipment and piping operating at 95 °F (35 °C) to 130 °F.
External carbonate cracking of buried gas transmission pipelines is well known. Cathodic protection raises the pH of the electrolyte beneath disbonded coating on the outside of a pipeline. CO2 in the soil from decaying organic matter readily dissolves in the high-pH electrolyte and forms a concentrated mixture of carbonate and bicarbonate in the pH range of 8 to 11. It is this solution that causes external IGSCC in the potential range of –0.5 to –0.8 V vs. SCE. Cracking is worse close to compressor stations where the temperature is near 150 °F (65 °F) but takes longer at temperatures below 100 °F. The range for cracking is reported to be 50 °F (10 °C) to 140 °F (60 °C).
Conclusions
• Leaks in shale gas pipelines were caused by IGSCC.
• The agent responsible was spent H2S scavenger, which accumulated at low points in the pipeline–initially as MEA plus dithiazine and ultimately as solid amorphous dithiazine plus MEA.
• CO2 from the process gas readily dissolved in the high pH liquid forming a mixture of carbonate and bicarbonate that caused IGSCC at regions of high residual stress close to girth welds.
• Cracking due to spent H2S scavenger is a variant of carbonate cracking reported in refinery amine units, FCCU and external carbonate cracking in buried pipelines.
Mitigation
• Change the H2S scavenger or prevent the spent scavenger from entering the flowline by using a knockout system.
• Limit the pH to <8.
• Perform a PWHT on scavenging equipment.
• Frequently pig and flush existing pipelines.
• Risk-rank lines based on severity of consequences at the location of low points, and shut in those where the risk exceeds risk tolerability criteria.
• Apply fitness-for-service assessment API 579-1, Paragraph 9.5.2—Leak before break analysis.8
• Implement a leak detection program.
• Apply mitigation for leaks and check values to reduce lost inventory.
• The use of fracture mechanics to determine critical crack size for rupture and remaining life was limited because present crack size, crack growth rates, and interaction between adjacent cracks were all unknown.
• Determine if the general corrosion rate will increase if dithiazine (a good corrosion inhibitor) is absent.
†Trade name.
References
1 API 5L, “Specification for Line Pipe” (Washington, DC: API, 2013).
2 API 1104, “Standard for Welding Pipelines and Related Facilities” (Washington, DC: API, 2013).
3 ANSI/NACE MR0175/ISO 15156, “Petroleum and natural gas industries—Materials for use in H2S-containing environments in oil and gas production” (Houston, TX: NACE International, 2015).
4 J. Gutzeit, J.M. Johnson, “Stress Corrosion Cracking of Carbon Steel Welds in Amine Service,” CORROSION/86, paper no. 206 (Houston, TX: NACE International, 1986).
5 P.G. Hughes, “Stress Corrosion Cracking in an MEA Unit Case Study,” UK National Conference (1982), p. 87.
6 API 945, “Avoiding Environmental Cracking in Amine Units” (Washington, DC: API, 2003).
7 R.N. Parkins, R.R. Foster, “Line Pipe Stress Corrosion Cracking,” CORROSION/86, paper no. 320 (Houston, TX: NACE, 1986).
8 API 579-1/ASME FFS-1, “Fitness-For-Service, Third Edition” (Washington, DC: API, 2016).
Bibliography
Bagdasarian, A.J., C.A. Shargay, and J. Coombes. Oil Gas J. 1, 13 (1992): p. 42.
Beavers, J.A., N.G. Thomson, and R.N. Parkins. “Stress Corrosion Cracking of Low Strength Carbon Steels in Candidate High Level Waste Repository Environments.” Environmental Effects Nuclear and Chemical Waste Management 5 (1985): pp. 279-296.
NACE Publication 34108, “Review and Survey of Alkaline Carbonate Stress Corrosion Cracking in Refinery Sour Waters.” Houston, TX: NACE International.
Richert, J.P., A.J. Bagdasarian, and C.A. Shargay. “Extent of Stress Corrosion Cracking in Amine Plants Revealed by Survey.” Oil Gas J. 6, 5 (1989): p. 45.
Rivera, M., S. Bolinger, and C. Wollenweber. “Carbonate Cracking Risk Assessment for a FCCU Gas Plant.” CORROSION 2004, paper no. 04639 (Houston, TX: NACE, 2004).
Treseder, R.S. “Influence of Yield Stress on Anodic Stress Corrosion Cracking Resistance of Weldable Carbon and Low Alloy Steel with Yield Strength Below 100 ksi.” Welding Research Council, WRC Bulletin 243, Part 2. 1978.