This article presents the life extension approach to maintain safe operation of an aging offshore wet crude pipeline. The approach includes the evaluations of corrosion threats, pipeline integrity, and effectiveness of corrosion control practices.
The external corrosion activities were primarily attributed to ineffective cathodic protection due to shielding by disbonded coating. In addition to water cut, low flow rate promoting water and solids accumulation was determined to be the main driver for localized internal corrosion.
Based on the integrity assessment, it was concluded that the pipeline is fit for service to be continued in operation, provided that the recommendations are implemented.
Offshore hydrocarbon exploration started as early as the 1850s when the first drilling was carried out in California, USA. While the Gulf of Mexico started with oil first being produced in 1938, the North Sea was first explored as a potential offshore area in the early 1960s. Since then, the pace of oil exploration and production in shallow water has gradually increased to deep water.1
Besides corrosion, the pipelines are also exposed to hazards like extreme weather conditions, collision with vessels, trawl impact, and pipeline span. The Committee on the Safety of Marine Pipelines reported major causes of subsea pipeline failures in the USA, identifying (internal and external) corrosion as the highest threat (48%), followed by other hazards (26%), maritime activities (14%), and natural forces (12%).2
Costs for managing corrosion represents a significant portion of operating expenses in the offshore industry, especially for aged pipelines. Different risk approaches might be designed and used to minimize these costs and to direct the efforts to improve reliability and availability of the structure.1-2
Safeguarding the structural integrity of subsea pipelines throughout their entire lifetime is not always easy. This can be more challenging beyond original design lifetime. This article presents the results of a comprehensive study performed for life extension of an aging 42-year-old critical offshore pipeline, thereby providing necessary assurance for safe continued operation. This is a 48-in (1.22-m) pipeline handling degassed crude oil with 8 to 10% water cut.
Life Extension Study Approach
The life extension study approach included the following:
- Mechanical integrity
- Internal corrosion
- External corrosion
Mechanical Integrity
Mechanical integrity assessment includes the evaluation of pipeline corroded conditions resulting from corrosion through fitness for service (FFS) analysis. The maximum allowable operating pressure (MAOP) may have to be reduced to avoid the leak or risk of rupture.
The FFS assessment was performed using material properties, and operating and inspection data generated from inline inspection (ILI) that applied the magnetic flux leakage (MFL) technique to detect corrosion and structural anomalies.
ASME B31G, “Manual for Determining the Remaining Strength of Corroded Pipelines,”3 provides four evaluation methods, from the most conservative Level 1 to the complex, data-intensive Level 4 for assessing the remaining strength of a corroded pipeline designed in accordance with ASME B31.44 and ASME B31.85 for liquid and gas pipelines.
The evaluation can result in small pits with severe metal loss passing the assessment whereas large anomalies with minor metal loss fail the assessment. The guideline uses the Estimated Repair Factor (ERF), which is the ratio of the pipeline MAOP to the Predicted Failure Pressure, to prescribe the safe operation. Corroded areas with ERF ≥1 shall be repaired immediately and ERF ≥0.95 requires careful monitoring if the corrosion activity is not completely arrested.3
Furthermore, the impact of the following threats should be addressed:
- Concrete coating damage
- Free spans exceeding allowable length
- External damages (e.g., dents, cracks, gouges, bending, etc.)
- Crossings status
Internal Corrosion
The internal corrosion assessment included the following evaluations:
- Corrosion Severity Predictions: The type of damage mechanisms and corrosion severity of a system depend on fluid compositions, the presence of corrosion species (i.e., water, gases, organic acids, solids, and microorganisms), and flow regime. In this case, the corrosion rates were determined by using Multicorp† and CORLIS† as well as the ILI data.
- Corrosion Control Review: Effectiveness of existing corrosion control practices (i.e., corrosion inhibition and scraping) was reviewed.
External Corrosion
The external corrosion assessment included the following reviews:
- Coating: As the primary external corrosion control measure, the coating condition was assessed using subsea inspection survey reports, and leak, repairs history, and ILI reports.
- Cathodic Protection (CP): Galvanic anodes were installed along the pipeline to supplement the protective coating as external corrosion control measures.The CP condition was reviewed based on data availed through the subsea inspection survey reports.
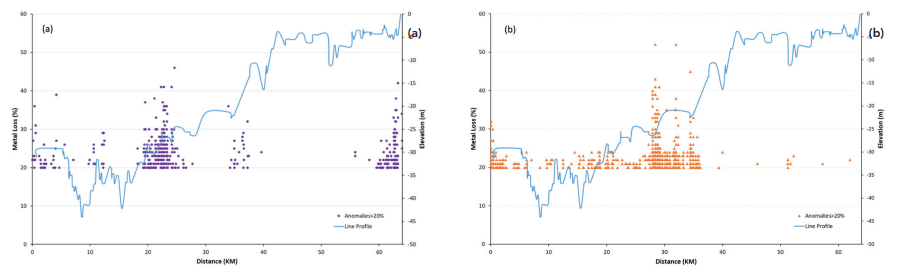
Results and Analysis
Mechanical Integrity
Based on ILI results, the external and internal corrosion anomalies were more densely distributed at the segments between KP 18 to 28 and KP 60 to 64 [Figure 1(a)], and the low elevation sections until 35 km [Figure 1(b)], respectively.
The curve generated from the FFS assessment of the 48-in pipeline is shown in Figure 2, which demonstrates safe operation at the MAOP without repair. However, considering the criticality of this pipeline, locations where metal loss exceeded 50% were sleeved.
The pipeline stability is not impacted by the concrete weight coat damages revealed through external subsea inspection reports except isolated sections, which were mitigated with concrete mattresses.
Internal Corrosion
The pipeline carries sweet crude with maximum carbon dioxide (CO2) content of 0.42 mol% with 8 to 10% water cut. The internal corrosion anomalies in the first ~35 km section, as shown in Figure 1(b), were attributed to water accumulation and potential microbes coupled with insufficient treatment and cleaning.
The oil-water flow pattern is important in determining the internal corrosion risk. Wetting behavior of the water phase and corrosion rate are affected by other factors, such as water chemistry, type of oil, additives, flow regime, fluid velocity, and surface condition of the pipe wall.6-7
An investigation on a Saudi Arabian crude confirmed that intermittent wetting is dominant at oil-water mixture velocities lower than 1.5 m/s and water cuts less than 10%. Water separation occurs when the water cut is higher than 10%.
At the same oil-water mixture velocity, increasing the water cut leads to a higher possibility for the coalescence of water droplets and the oil phase does not carry sufficient turbulent energy to disperse the water phase. Stable oil-water stratified flow exists at low velocities, although there is still some mixing at the oil-water interface.6
Full water entrainment occurs when the oil-water mixture velocity is higher than 1.5 m/s and water cut is lower than 10%. In this case all the water phase flows as water droplets dispersed in the oil phase. In this oil-water mixture velocity range, increasing the water cut leads to a possibility for intermittent phase wetting and corrosion (Figure 3).
It should be noted that the oil type has a significant effect on the wetting behavior and corrosion; some of the effects can be readily explained by the differences in their physical properties.6-7
Corrosion simulation using Multicorp software predicted a general corrosion rate of 8.6 mpy with stratified flow. Low superficial mixture velocity of 0.13 m/s promotes water and solids accumulation; thus, it increases the potential of underdeposit corrosion and microbiologically influenced corrosion. CORLIS predicted the latest ILI anomalies growth and calculated what the pitting rate and wall thickness would be in 2030.
The results indicate that maximum pitting rate will be 11 mpy with average 30% wall thickness loss in 2030 (Figure 4). The corrosion control of this pipeline includes satisfactory cleaning scraping, and adequate dosage of corrosion inhibition. However, the system does not have corrosion monitoring and sampling points to check the corrosion inhibition effectiveness and availability. There was no monitoring on the microbial level inside the pipeline.
External Corrosion
External coatings for the onshore and offshore sections of the pipeline are tape wrap and bitumen wrap covered by concrete weight coat, respectively. Although the CP readings showed a protected condition, the external corrosion anomalies revealed in Figure 1(a) have indicated deficiencies in the control measures. Both systems are CP-shielding coatings.
When disbondment or blistering occurs, such coatings divert the current from its intended path. Therefore, the CP current that is applied per design cannot adequately protect the external pipeline surfaces as intended.
The challenge is that CP shielding due to loss of adhesion for the coating cannot be identified reliably. ILI data can show local concentrations, or “hot spots,” and these can be defined on the basis of pit depth (i.e., a concentration of relatively deep pits within a short segment of line), or corroding area.9
Most coating authorities consider a coating’s life to first maintenance depending upon the coating type and coating exposure conditions severity. Coating integrity is compromised by the interaction with environmental elements, which may degrade the coating surface, permeate into the coating, and/or penetrate through the coating to affect the underlying substrate.8
Tape coatings applied with a minimal overlap are prone to adhesion loss and separation at the overlap. Corrosion risk at the overlap can be diagnosed from potential surveys but is challenging. This failure mode is commonly accompanied by more extensive adhesion loss and CP shielding.
Offshore pipelines with bitumen wrap are also susceptible to the CP shielding effect, but with a lesser severity. In addition, the bitumen offshore section with concrete weight coating is also susceptible to microbial degradation and axial expansion.
Conclusions
This study provided comprehensive life extension assessment of a 42-year-old subsea crude oil pipeline. The assessment involved a wide spectrum of technical disciplines such as piping, materials, corrosion, coating, and CP.
The assessment concluded that the pipeline is safe to operate provided corrective actions and proactive measures are undertaken to ensure reliable operations. These actions include the following:
- Utilize an advanced ILI technology capable of detecting and sizing small anomalies to overcome the limitations with the currently utilized ILI technique.
- Perform yearly ILI to monitor external and internal corrosion. To date, there is no reliable ILI inspection technology currently available to detect and map external coating disbondment.
- Establish a pipeline repair readiness plan.
- Install online probes to gauge the effectiveness of corrosion inhibition.
- Monitor the microbial level in the pipeline through either serial dilution or quantitative polymerase chain reaction (qPCR). If the corrosion causative microbes such as sulfate reducing bacteria and SRA levels are greater than or equal to 103, then biocide treatment should be designed.
The life extension has avoided substantial replacement cost.
† Trade Name.
References and About the Authors